Abstract
Background: Clonal hematopoiesis of indeterminate potential (CHIP) refers to the presence of a hematopoietic clone with a common leukemia driver mutation without diagnosis of an underlying hematopoietic disease. The prevalence of CHIP is increasing with age and is associated with pro-inflammatory states, higher risk of cardiovascular diseases (CVD) and therapy-induced leukemia. However, these CHIP-associated risks overlap with treatment-related toxicities of breast cancer therapy, which potentially supports the integration of CHIP into treatment- and survivorship plans. However, so far no data on the feasibility and acceptance of a CHIP-based aftercare are available. The aim of this pilot trial is to evaluate the feasibility to integrate pre-treatment CHIP diagnostics into the routine care of older breast cancer patients.
Materials and Methods: 80-100 patients with limited stage breast cancer aged ≥ 60 years without known hematological disease will be included. CHIP is assessed by targeted next generation sequencing from peripheral blood samples. The primary outcome measures the estimation of willingness to participate. Secondary outcome measures include evaluation of patient acceptance of the study process, potential fears in relation to CHIP-positivity, and cardiovascular risk profile of CHIP-positive versus CHIP-negative patients.
Conclusions: In case this study meets its primary endpoint, the results are used to design a larger cohort study that integrates an intensified CHIP-tailored survivorship program, in order to minimize late treatment-related toxicities and improve long-term outcomes of older breast-cancer patients.
Clinical trial registration number: German Clinical Trials Register (Deutsches Register für klinische Studien, DRKS) DRKS00031021.
Keywords
1. Introduction
1.1 Background
With 46,000 new cases, breast cancer is the most common solid cancer in women ≥ 65 years in Germany[1]. Especially when diagnosed at early stages, the long-term outcomes are excellent[2]. Thus, the balance between long-term control of breast cancer and treatment-related toxicities is of utmost importance. Clonal hematopoiesis of indeterminate potential (CHIP) confers to the presence of a hematopoietic clone carrying a hematological malignancy-associated mutation without an underlying hematological malignancy[3]. The incidence of CHIP is rising with age[4,5]. CHIP is associated with an increased risk of (therapy-related) myeloid neoplasms[6-9], and cardiovascular events[4,10]. As several of CHIP-associated risks overlap with known long-term toxicities of breast cancer therapy, it seems indispensable to understand its interplay and to integrate CHIP into treatment and survivorship concepts of older adults with breast cancer. However, so far no data on the implementation of a CHIP-based strategy into survivorship care are available. Thus, incorporation of CHIP into real-world medicine needs to be established and evaluated. These requirements led to the concept of Crunchy-CHIPs (Cardiovascular and haematological survivorship in breast cancer patients at high risk caused by clonal hematopoiesis of indeterminate potential-study).
1.1.1 CHIP: definition and general aspects
The most abundant definition of CHIP, that was recently included in the 2022 World Health Organization (WHO) classification of myeloid malignancies[11] is the presence of a hematopoietic clone carrying a hematological malignancy-associated mutation with a variant allele frequency (VAF) of ≥ 2% without otherwise unexplained cytopenias[3]. Despite that, much smaller VAFs were described depending on the depth of sequencing and the number of sequenced genes[5,12]. At least in some circumstances (e.g., risk of acute myeloid leukemia (AML) related to Tumor Protein 53 (TP53) and isocitrate dehydrogenase (IDH) -mutations[13], or mutations in DNA damage repair (DDR) genes[14]), these smaller VAFs seem clinically relevant, as they provide a fitness advantage and can expand easily[13].
CHIP occurs most commonly in genes of the epigenetic modifiers DNA methyltransferase 3 alpha (DNMT3A), Tet methylcytosine dioxygenase 2 (TET2), and Additional Sex Combs-Like 1 (ASXL1) ('DTA mutations')[4,5]. Besides, common mutations affect IDH1/2, spliceosome genes, and DDR genes, such as TP53, and Protein Phosphatase, Mg2+/Mn2+ Dependent 1D (PPM1D)[4,5,13,15]. Of note, the spectrum of CHIP mutations that were studied in different trials was not standardized; thereby, the variance in CHIP incidence between different data sets is easily explained.
Although CHIP was recently described to appear as early as in utero at very small VAFs[16], its incidence strongly increases with age, affecting at least 10% of individuals ≥ 60 years[4]. The CHIP incidence in breast cancer patients might differ depending on age and prior exposure to genotoxic agents which includes a smoking history. Albeit, the CHIP incidence in women with breast cancer ≥ 70 years was described as high as 44%[17]. CHIP is associated with an increased overall mortality in adults ≥ 70 years[4].
Most of the mutations confer to a pro-inflammatory phenotype, which is based on pre-clinical[18-20] and clinical data[10,21]. As a clinical surrogate marker, high-sensitive C-reactive protein (hsCRP) was increased in CHIP-positive individuals[22]. Furthermore, other cytokines, such as interleukin-6 (IL-6)[23,24] or interleukin-8 (IL-8)[10] were also shown to be elevated. As a consequence, it is not surprising that the presence of CHIP fuels diseases like chronic obstructive pulmonary disease[25,26], liver cirrhosis[27], autoimmune diseases[28], and infections, such as Clostridium difficile enterocolitis and severe COVID-19 infection[29] (Figure 1), most likely via a pro-inflammatory crosstalk.
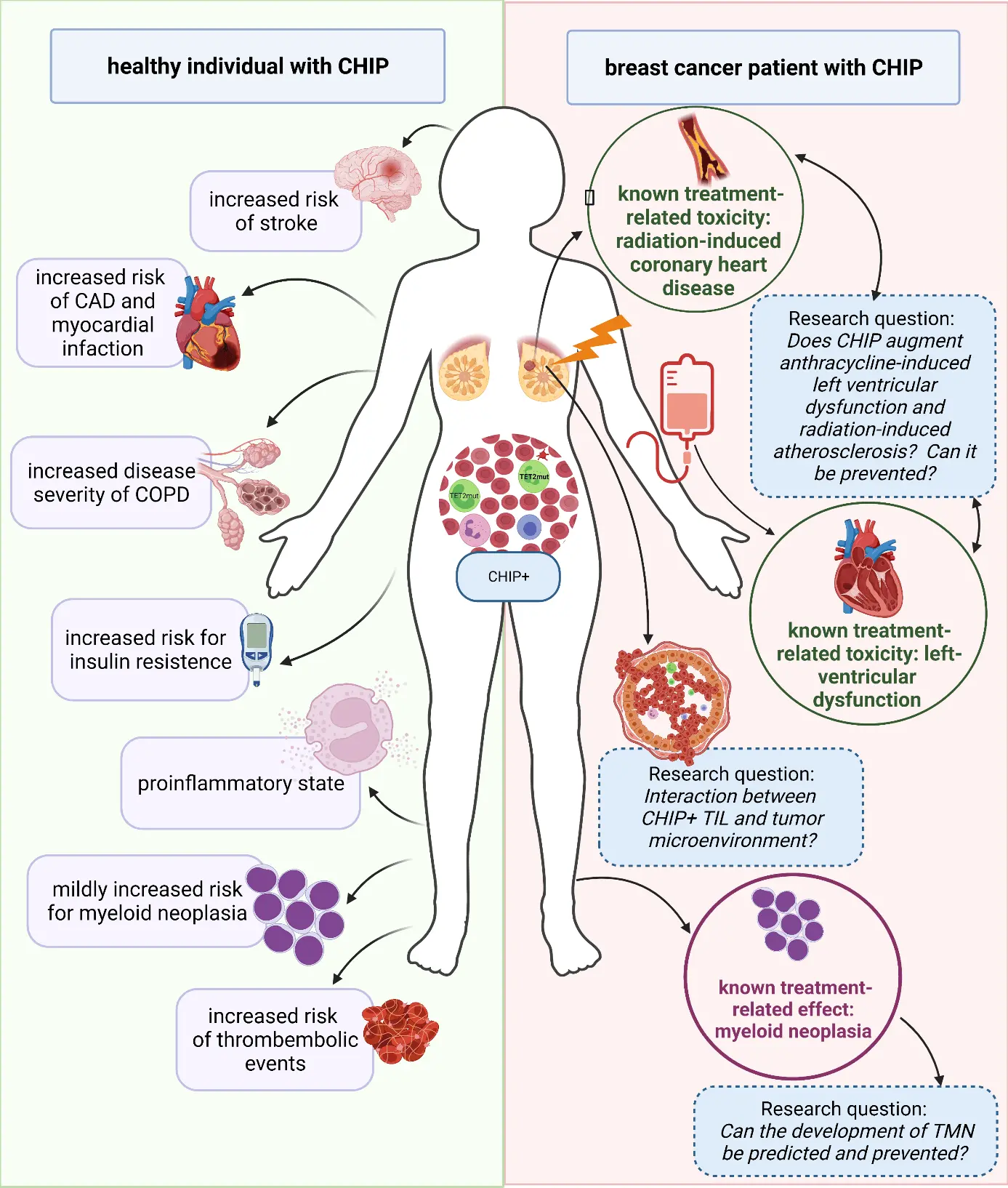
Figure 1. CHIP-associated risks in healthy older individuals, breast cancer treatment-related toxicities, and their suspected interplay with CHIP. CAD: coronary artery disease; CHIP: clonal hematopoiesis of indeterminate potential; COPD: chronic obstructive pulmonary disease; TIL: tumor-infiltrating leukocytes; TMN: therapy-related myeloid neoplasia.
1.1.2 The interplay between CHIP and solid cancers
CHIP is frequently found incidentally when peripheral blood is used as comparator for mutational profiling of solid cancers[30,31] and acts as a confounder in liquid biopsies[32,33]. Recently, infiltration of the tumor microenvironment by CHIP-positive tumor-infiltrating leukocytes was described[34]. This prompts the question whether the pro-inflammatory properties of CHIP-positive leukocytes modify the TIL-tumor interactions and promote the tumor growth. This is not sufficiently studied yet but first results in an experimental model of colon cancer with the experimental equivalent for DNMT3A-CHIP showed increased infiltration of colon cancer by immune cells and, in preliminary data, a decreased phagocytic capacity[35], suggesting a permissive microenvironment. Given the known tumor-promoting effect of chronic inflammation, it could be expected that CHIP acts as a tumor-promoting factor. First data that support such a negative impact showed an increased mortality due to progressive solid cancers in CHIP-positive patients[6]. This needs to be confirmed in larger data sets and tumor-entity specific analyses. With regard to breast cancer, CHIP could further influence tumorigenesis and tumor promotion via the cancer-metabolic interplay. Metabolic syndrome and its components (diabetes, dyslipidemia, and obesity) are well-known risk factors, especially for hormone-receptor positive breast cancer in postmenopausal woman[36]. Although the pathophysiology behind this risk is complex and multifaceted, it is largely determined by the chronic inflammatory and endocrine properties of the adipose tissue[37]. Thus, CHIP comes again into play with its pro-inflammatory nature and its interacting role within the metabolic axis. Specifically, TET2-Chip has been shown to potentiate age- and obesity-related insulin resistance in a diabetic mouse model that can be modified via inhibition of the inflammasome[38]. Whether a reduction of CHIP-related inflammation and/or a consequent control of metabolic disturbances can abrogate the tumor promotion remains elusive and will be a vibrant research question for the next years as it provides a promising target for cancer prevention together with cardiovascular risk reduction.
Besides the possible CHIP-cancer interplay, CHIP could have a very practical impact on therapy-related toxicities during cancer therapies which is largely understudied so far. With regard to therapy-induced cytopenia during chemotherapy, data are lacking although CHIP has been shown to be associated with a delayed time to neutrophil reconstitution after autologous stem-cell transplantation[39].
1.1.3 Treatment-related toxicities after breast cancer therapy: therapy-related myeloid neoplasms
Therapy-related myeloid neoplasms (TMN) after breast cancer therapy are rare, as they occur in ~0.5% of patients within 10 years of chemotherapy-including treatments[40] but their outcomes are grim with a 3-year overall survival of ~30%[41], even after allogeneic stem-cell transplantation. CHIP is related to the exposure with genotoxic agents and smoking[9] and has been identified as a predisposing factor for TMN[6-9]. On a mechanistical basis, pre-existing CHIP clones might own a growth advantage upon genotoxic stress and can acquire secondary mutations that lead to leukemic transformation of these clones. In addition, genotoxic therapy can induce mutations that lead to the development of CHIP; later, secondary acquisition of further mutations initiates a leukemic transformation. These two potential mechanisms leading to TMN are depicted in Figure 2. These hypotheses are underlined by data from the MSK-IMPACT study[9]. In this, CHIP clones that harboured a mutation in the DNA damage genes TP53, Checkpoint kinase 2 (CHEK2), or PPM1D showed an increase in allelic burden during chemo- or radiation therapy[9] as a sign for a fitness advantage upon these respective therapies. In 59% of patients who developed a TMN, at least one leukemia driver mutation that was present in TMN could be detected as CHIP prior to initial therapy[9]. This is underlined by the findings from the Woman Health Study, in which 100% of participants with CHIP in TP53 or IDH1/2 developed spontaneously a myeloid neoplasia over time[13]. However, it should be noted that even analyses of large cohorts include only small numbers of individuals with a respective mutation, thus, a detailed risk assessment to predict the development of (T)MN remains an unmet clinical need. In the context of early breast cancer care, this could become a highly relevant topic in future if the risk of TMN is weighed against the risk reduction of cancer recurrence by adjuvant chemotherapy. Thus, depending on the individual risk, adapting the (neo-)adjuvant therapy might provide the necessary balance of risks, but needs thorough evaluation before such as approach is integrated into clinical routine.
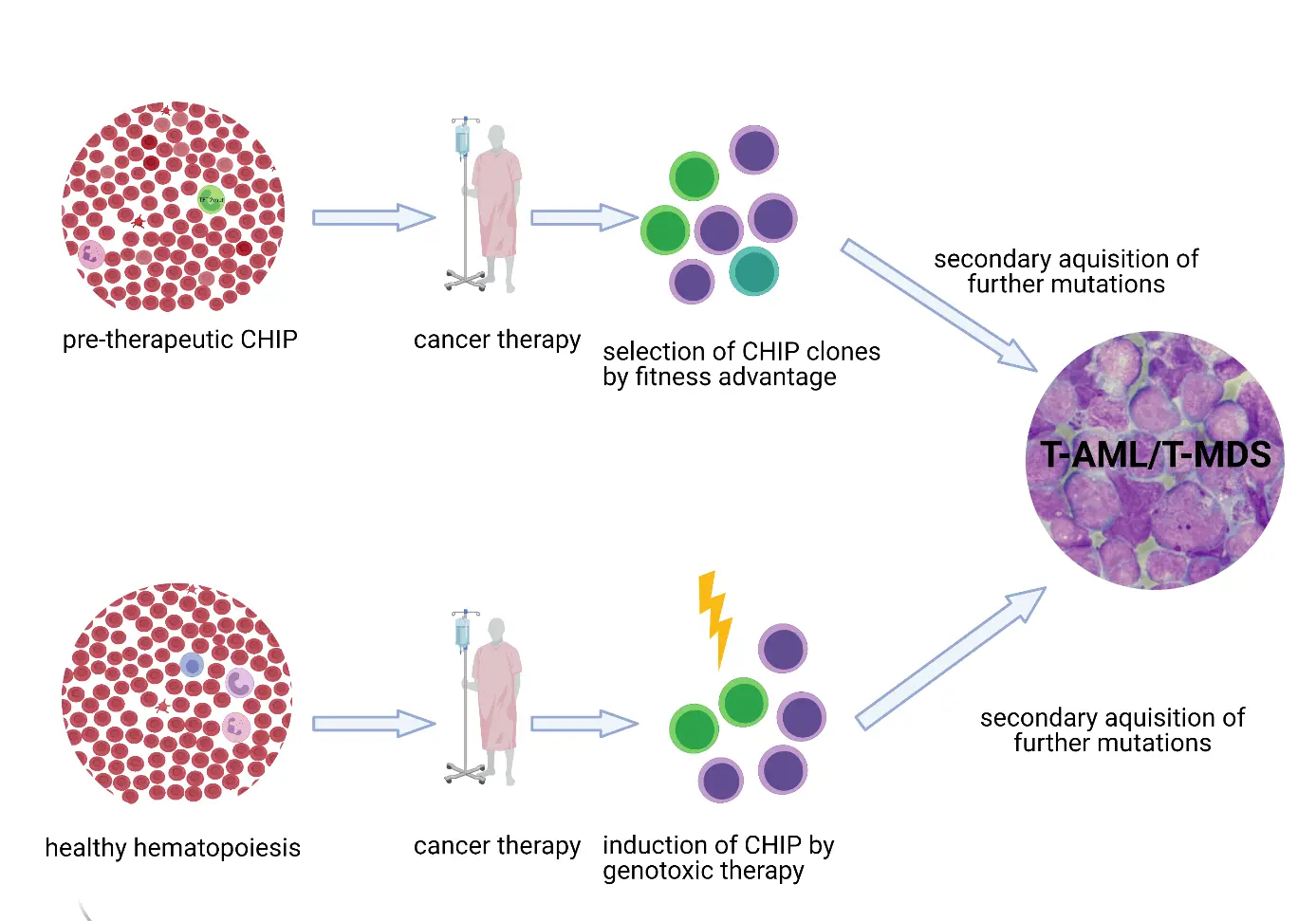
Figure 2. Possible pathways to therapy-related myeloid neoplasms in relation to CHIP. By mechanistical view, CHIP can pave two pathways to the development of therapy-related myeloid neoplasms: Firstly, in an individual with pre-existing CHIP, the application of cytotoxic chemotherapy can lead to a selection of CHIP clones with fitness advantage. Further acquisition of mutations by genotoxic therapies can finally cause transformation into a myeloid neoplasm. Secondly, in an individual without pre-existing CHIP, genotoxic therapy can induce CHIP clones which can advance into myeloid neoplasms after further acquisition of mutations. T-AML: therapy-related acute myeloid leukemia; T-MDS: therapy-related myelodysplastic syndrome; CHIP: clonal hematopoiesis of indeterminate potential.
1.1.4 CHIP and the risk for cardiovascular diseases
CHIP was shown to increase the overall mortality in the general population, which was not caused by an increased leukemia-induced death-rate but increased cardiovascular (CV) events[4]. Further, CHIP was demonstrated to be associated with an increased risk for coronary artery disease (CAD)[4], peripheral artery disease[42], and stroke[4,43,44](Figure 1).
Recent work has elucidated the pathophysiological mechanism of the promotion of arthrosclerosis by CHIP: Based on the atherosclerosis-prone, low-density lipoprotein receptor-deficient (Ldlr -/-) mouse model combined with competitive Tet2-deficient hematopoietic stem-cell (HSC) transplantation that resembles TET2-CHIP, Tet2-deficient macrophages were shown to have an increased NOD-, LRR- and pyrin domain-containing protein 3 (NLRP3) inflammasome–mediated secretion of interleukin-1ß (IL-1ß) after stimulation with Lipopolysaccharide and interferon-gamma. In addition, other pro-inflammatory cytokines were also upregulated[18]. This IL-1ß secretion is potentially the most potent causative link to the accelerated atherosclerosis as it orchestrates low-grade inflammation of endothelial cells and enhances proliferation of smooth muscle cells[45]. Thus, it is not surprising that inhibition of NLRP3 inflammasome was demonstrated to reverse the pro-atherosclerotic effect of Tet2-deficiency in the same experimental model[18]. In concordance, an exploratory analysis from the Canakinumab Antiinflammatory Thrombosis Outcome Study trial demonstrated a reduction in major adverse cardiac event (MACE) for patients receiving the neutralizing anti-IL-1ß antibody canakinumab (hazard ratio (HR), 0.38 [95% confidence interval (CI), 0.15-0.96]) in comparison to placebo-treated patients[46]. This is further underlined by a recent analysis of a cohort of ST segment elevation myocardial infarction patients. Among these, patients with DNMT3A/TET2-CHIP experienced an increased rate of death (30.9% vs. 15.5%, P = 0.001) and MACE (44.5% vs. 21.8%, P < 0.001) during a medium follow up of 3 years[24].
Of interest, the relationship between CHIP and atherosclerosis is most likely not only unidirectional as an enhanced proliferation of HSCs in the presence of atherosclerosis was recently suggested, presumably due to increased inflammation and hyperlipidemia[47]. Although CHIP was shown to act as CV risk factor independent of established risk factors, such as smoking, or hyperlipidaemia[4], it remains elusive whether CHIP confers any substantial CV risk in individuals without additional risk factors, such as dyslipidemia, smoking, or hypertension, as most of the studied cohorts included many metabolic high-risk individuals (e.g., diabetes)[4]. In line, the seminal work in the atherosclerosis-prone mouse model resembling TET2-CHIP only demonstrated an accelerated plaque formation upon high fat/cholesterol diet, whereas mice fed with normal diet did not show this acceleration[18]. This suggests the interplay of traditional CV risk factors with CHIP/pro-inflammatory state as necessary requisite for atherosclerosis acceleration rather than a causative role of CHIP as single risk factor. As a consequence, reduction of CHIP-induced CV risk might be achieved by consequent control of established risk factor or require additional anti-inflammatory treatment. As this question is crucial for future management and reduction of CHIP-related CV risk, this needs to be determined in different patient cohorts, most likely with an additional focus on early endothelial dysfunctions.
Besides the increased risk for CAD, CHIP was demonstrated to increase the risk for heart failure[48,49]. This finding is further supported in experimental mouse models resembling TET2-[50] and Janus kinase 2-[51] CHIP. Furthermore, CHIP with DNMT3A- or TET2-mutation was shown to be independently associated with an accelerated heart failure progression in terms of death and/or heart failure hospitalization (DNMT3A : HR, 4.50; 95% CI, 2.07-9.74; P < 0.001, TET2 : HR, 3.18; 95% CI, 1.52-6.66; P = 0.002) irrespective of the underlying ischemic/non-ischemic etiology[49].
1.1.5 Treatment-related toxicities after breast cancer therapy: cardiovascular sequelae and cardio-metabolic interplay
Many components of breast cancer therapy carry a risk for cardiovascular side-effects and sequelae, such as anthracycline-induced left ventricular dysfunction (AILVD)[52,53], radiation-induced atherosclerosis[54], taxane-related early endothelial dysfunctions[55], or the prothrombotic properties of tamoxifen[52] (Figure 1). Given the role of cardio-metabolic risk factors for the development of breast cancer, it is not surprising that breast cancer patients show an increased prevalence of cardio-metabolic risk factors, even at baseline[56,57] that further predispose to such treatment-related toxicities[53,54].
As CHIP is in between this cardio-metabolic interplay and confers an increased CV risk, as described above, it might have a further impact on these toxicities during treatment and survivorship (Figure 1). First data support this view: In lymphoma patients receiving anthracyclines, TET2-CHIP was associated with an increased rate of AILVD[58]. Furthermore, in AML patients treated with intensive anthracycline-containing regimens, the presence of CHIP-mutations was associated with an increased risk for CV events (HR, 1.74; 95% CI, 1.03-2.93; P = 0.037)[59]. The same accounted specifically for TP53 (HR, 4.18; 95% CI, 2.07-8.47; P < 0.001) and ASXL1 (HR, 2.37; 95% CI, 1.21-4.63 P = 0.012) mutations[59]. These data are supported in a mouse model of AILVD with underlying TP53 -CHIP[60]. Should this be further confirmed, intensification of primary preventive measures to mitigate toxicities, or even adjusting the treatment according to the individualized risk might be advantageous although this is still a long way off.
During breast cancer survivorship, the most common causes of death are CV events, including CAD and heart failure[61-67]. Data from childhood cancer survivors suggest that traditional CV risk calculation in this population underestimates the risk[68], which was also demonstrated for the use of Framingham risk calculation in adult breast cancer patients[69]. In part, that can be related to the increase in cardio-metabolic risk factors after breast cancer therapy[56,70]. Whether CHIP-positive patients carry an even higher cardio-metabolic risk during survivorship that further impacts these events, is unknown, but appears likely given the multifaceted interplay between these risks. Another open question is, whether CHIP-positive patients require an intensified survivorship program, including stringent reduction of traditional CV risk factors or even an anti-inflammatory approach.
1.1.6 Genetic counselling in older adults
Although CHIP diagnostics do not refer to germline but somatic mutations, it is a kind of acquired predisposition for leukemia and CV conditions; thus, it represents a form of predictive genetic testing. This is especially important as no explicit recommendations for individualized prevention exist in case of positivity. Predictive genetic testing can potentially result in psychological adverse reactions, such as anxiety, or distress, which might be dependent on the perception of controllability of the respective condition[71]. Despite, a recent systematic review revealed mostly no serious negative impact of genetic testing for CV diseases and cancer on quality of life (QoL), anxiety, or distress[71]. As several hereditary cancer syndromes or cardiovascular diseases tend to have an early onset in life, studies are focussed on younger adults, although older adults were shown to be willing to participate in genetic testing[72]. Despite, acceptance and psychological distress of predictive genetic testing is understudied in older adults.
A recent survey among survivors of breast cancer at young age on preferences and attitudes towards CHIP testing revealed an interest in CHIP testing in > 75% of participants after receiving some education on this condition. 70.8% expressed that they would expect knowing about CHIP positivity would help them to change their behaviour, in order to reduce associated disease risks. Despite that, 22.7% stated hypothetically that learning that they would be CHIP positive would be "more than [they] could handle emotionally"[73]. Notably, 92% of participants had an educational level of college or above and the median age was 46 years (range: 41-54 years)[73]. Although these results are very informative about patient preferences with regard to CHIP testing, the older age group which is mainly affected by CHIP, was not included into the survey. As a consequence, we see a particular need to assess patient acceptance of CHIP evaluation during our pilot trial.
1.1.7 Study rationale
Given the pro-inflammatory properties of CHIP and its suspected role within the (hemato-) cardio-metabolic crosstalk, CHIP will likely gain an important role within geriatric oncology[74], especially in a patient-centred individualized survivorship care that takes into account the individual risks for cardiometabolic diseases and TMN. Hitherto, effects of CHIP are almost exclusively studied in retrospective data sets. Integration in routine clinical care is not systematically performed and evaluated, and the acceptance by patients of such an approach is unknown. In this pilot trial, we determine the feasibility of integrating CHIP diagnostics into the routine work-up during breast cancer diagnostics and treatment initiation. A main goal of this piloting is to assess the willingness to participate, the prevalence of CHIP and cardiovascular risk factors, and overall patient satisfaction in our cohort in order to determine sample sizes and potential measurements for a large CHIP-tailored survivorship trial in future.
2. Methods
2.1 Aims, study design, setting, and recruitment
2.1.1 Aims
Our study aims are as follows: 1) The primary aim is to estimate the willingness of participation with an accuracy of +/- 7.5%. 2) Secondary aims are to assess the patient acceptance of the study procedures and addition of CHIP diagnostics to the routine work-up, to evaluate the overall prevalence of pre-therapeutic CHIP in this cohort of breast cancer patients, to assess the feasibility to recruit 80-100 patients within 12 months, to assess the frequency of guideline-adherent cardiovascular preventive medications at baseline and after 12 months, and to characterize the prevalence of cardiovascular risk factors in CHIP-positive versus CHIP-negative patients.
2.1.2 Trial design and setting
80-100 patients will be recruited from the Essen University Hospital, Breast Cancer Center, Department for Obstetrics and Gynacology. The protocol was approved by the Institutional Ethics Committee, University Duisburg-Essen on May 18, 2022. The study is funded by a grant from the Medical Faculty of the University Duisburg-Essen. The trial is registered at German Clinical Trials Register (Deutsches Register für klinische Studien, DRKS), DRKS00031021.
2.1.3 Recruitment and consensus
A trial participation is offered to all patients ≥ 60 years presenting at our breast cancer center who are firstly diagnosed with breast cancer at a curative stage and further fulfil the eligibility criteria (Table 1). These eligibility criteria are very broad to avoid any selection bias. Written informed consent is obtained by all participants. The number of patients who reject participation is recorded without documentation of personal data.
Inclusion criteria |
Age ≥ 60 years |
Female gender |
First diagnosis of non-metastasized breast cancer (stage I-III) |
Must be able to understand written and spoken German |
Willing to comply with study procedures for the entire lengths of the study. |
Exclusion criteria |
Male gender |
Metastasized breast cancer |
Former history of acute myeloid leukemia, myeloproliferative diseases, or myelodysplastic syndrome |
Prior therapies with cytotoxic agents (excluding methotrexate for autoimmune diseases) |
Inability to give informed consent |
2.2 Study procedures
At baseline, pre-treatment blood samples will be collected. Patients undergo their planned breast cancer treatment (surgery, radiation therapy, hormone-/chemotherapy) without any modification (Figure 3). Study personnel will document cancer and treatment specific details. Approx. three months after diagnosis, results of CHIP diagnostics are discussed with patients at the hematological outpatient clinic. At this point in time, no additional laboratory values are taken. In case, the baseline assessment was incomplete (e.g., cardiac risk assessment), measures are completed. Patients who are CHIP-positive receive a written summary on associated risks, their general practitioner also obtain a summary of current evidence about risks for TMN and CV events together with the information that current preventive measures remain unchanged (Figure 3). Treating physicians are not blinded for CHIP results as treatment decisions are already determined at this point in time. Patients in whom one or more CHIP-mutations with high risk for TMN which we define currently as CHIP with mutations in the DNA damage pathway (including TP53, PPM1D, and CHEK2) are detected are offered a differential blood count and a hematology consultation every 3-6 months. The definition of high-risk CHIP can be adapted during the study if new data are published that suggest a modification. In case, CHIP occurs together with cytopenia, a standard work-up for cytopenia is offered, independent of the study procedures. If myelodysplastic neoplasia is excluded, this condition is termed clonal cytopenia of undetermined significance and further hematological surveillance according to the local standard-of-care is offered.
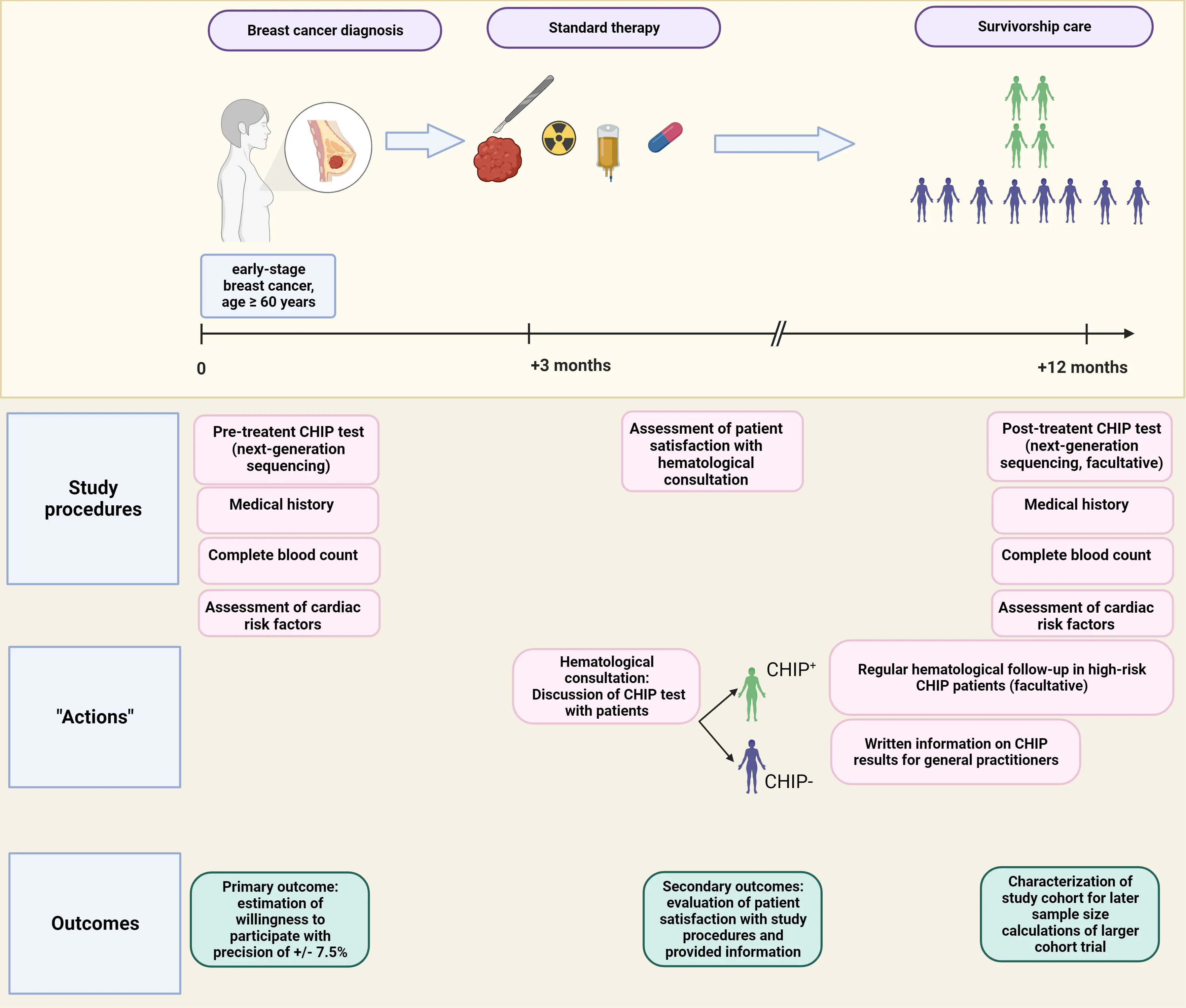
Figure 3. Overview on study procedures. CHIP: clonal hematopoiesis of indeterminate potential.
After the informative hematological consultation, patients are asked to answer the European Organisation for Research and Treatment of Cancer Quality of Life Questionnaire C30 (EORTC-QLQ-C30) questionnaire[75] together with a questionnaire on patient satisfaction and possibly associated fears with regard to CHIP diagnosis (Table S1). Another consultation is scheduled after 12 months; during that visit, another blood sample is collected and regular medication is recorded. An additional, posttreatment CHIP assessment is facultative at this point in time. Patients with high-risk CHIP mutations are offered to continue hematology consultations, for the other participants the trial ends after 12 months.
2.3 Measures
Measures will be obtained at baseline (T1), approximately three months after diagnosis (T2), and after 12 months (T3). Study measures are listed in Table 2.
Measure | Description | Time point |
Blood draw | CHIP analysis by NGS | Baseline, (+12 months, facultative) |
Cytokine panel (IL-1ß, IL-6, IL-18, c-peptide, leptin, adiponectine, IGF-1, iridin, soluble Klotho) | ||
Differential blood count, RDW, MCV | ||
Breast cancer-related data | Stage, grading, histological subtype | Baseline |
Treatment-related data | Intended treatment, accomplished treatment; treatment-related toxicities [acc. to CTCAE]: duration and severity of cytopenia, therapy-related infections, treatment modifications | During treatment |
CV risk assessment (lab) | hsTropI | Baseline, +12 months |
NT-proBNP | ||
total cholesterol | ||
LDL | ||
HDL | ||
hsCRP | ||
CV risk assessment (clin) | Score2 [76] | Baseline or +3 months |
BMI | ||
Waist circumference | ||
h/o arterial hypertension, as documented by antihypertensive medication or former diagnosis | ||
h/o + dx heart failure (HFREF, HFmrEF, HFpEF) | ||
h/o thromboembolic events (stroke, myocardial infarction, thrombosis, pulmonary embolism) | ||
h/o + dx CAD | ||
h/o + dx diabetes | ||
resting office blood pressure | ||
echocardiography (LVEF, E/A, E/E') | ||
h/o migraine with aura | ||
Family h/o MACE | ||
Medication history | ACE inhibitors | Baseline, +12 months |
ARBs | ||
ß-blockers | ||
CCBs | ||
statins | ||
APTs | ||
MRAs | ||
Anti-inflammatory drugs: NSAIDs, MTX, cholchicine, TNFa-blocker | ||
SGLT2 inhibitors | ||
GLP-1 receptor antagonists | ||
metformin | ||
DPP-4 inhibitors |
ACE: angiotensin-converting-enzyme; APT: anti-platelet therapy; ARBs: angiotensin receptor blockers; BMI: body mass index; CAD: coronary artery disease; CCBs: calcium channel blockers; clin, clinical; CV: cardiovascular; DPP-4: Dipeptidyl peptidase-4; IGF-1: insulin-like growth factor -1; GLP-1: glucagon-like peptide-1; hsCRP: high-sensitive c-reactive protein; hsTropI: high-sensitive troponine I; HDL: high density lipoprotein; HEFREF: heart failure with reduced ejection fraction; HFmrEF: heart failure with moderately reduced ejection fraction; HFpEF: heart failure with preserved ejection fraction; LDL: low-density lipoprotein; LVEF: left ventricular ejection fraction; MACE: major adverse cardiovascular events; MCV: mean corpuscular volume; MRA: mineralocorticoid receptor antagonist; MTX: methotrexate; NGS: next generation sequencing; NSAIDs: non-steroidal anti-inflammatory drugs; NT-proBNP: N-terminal prohormone of brain natriuretic peptide; RDW: red cell distribution width; TNFa: tumor necrosis factor alpha.
2.3.1 Blood sample collection and CHIP assessment
Blood will be drawn at baseline (pre-treatment), and after 12 months during a routine laboratory test. Approximately 4 ml of serum will be stored after centrifugation at -80 °C, peripheral blood mononuclear cells (PMBCs) will be collected and processed by BD Biosciences CPT vacutainer system according to the manufacturer's instructions and further stored at -80 °C.
Genomic DNA is extracted from PBMCs at baseline with QIAamp DNA Blood Mini Kit (Qiagen) and quantified by Qubit™ 1X dsDNA HS Assay Kit (ThermoFisher Scientific). 200 ng of genomic DNA will be subjected to next generation sequencing using Illumina NextSeq2000 at 2x 150 bp. A customized panel will be used (SOPHiA Genetics), covering 112 genes (Table S2). Data are processed with SophiaDDM Software (SOPHiA Genetics).
We will call genomic variants from the obtained sequence reads using the Snakemake[77] workflow dna-seq-varlociraptor (https://github.com/snakemake-workflows/dna-seq-varlociraptor), consisting of the following steps. Sequence reads will be mapped with BWA-mem[78], followed by conversion of polymerase-chain reaction duplicates into consensus reads with rust-bio-tools (https://github.com/rust-bio/rust-bio-tools) and base recalibration with GATK[79]. Next, the known CHIP-associated variants are called and the posterior allele frequency distribution of each variant is optained with Varlociraptor[80], followed by variant annotation with Ensembl Variant Effect Predictor[81], filtration with Vembrane[82] and false-discovery rate control with Varlociraptor. Finally, an interactive, portable visual report is generated using Datavzrd (https://github.com/datavzrd/datavzrd) and Snakemake.
In addition, a differential blood count, high-sensitive troponine I, N-terminal prohormone of brain natriuretic peptide (NT-proBNP), total cholesterol, low-density lipoprotein (LDL), high-density lipoprotein (HDL), and high-sensitive C-reactive protein (CRP) will be determined at baseline.
2.3.2 Clinical measures
Standard information on breast cancer stage, histology, treatment details, and measures of treatment tolerability are documented (Table 2). A detailed CV history and risk assessment is performed during outpatient visit at hematology department as listed in Table 2. QoL is assessed by EORTC-QLQ-C30 questionnaire together with patient acceptance evaluation (Table S1) after patient education about their CHIP status at hematology outpatient department.
2.3.3 Feasibility metrics
In order to assess the feasibility of integration CHIP diagnostics into routine care, we will collect several feasibility metrics. The enrolment rate (corresponding to the percentage of patients who are eligible and consented) will be documented, as well as the completeness of documentation (e.g., details of provided therapy, duration and severity of treatment-associated cytopenias during chemotherapy) as some patients receive parts of their therapy outside our center (e.g., radiation therapy), the retention rate (percentage of patients who complete also the 12-months follow-up), and the rate of sufficient sequencing results in ≥ 80% of samples.
In addition, patient satisfaction with information about the study and CHIP positivity itself and acceptance of procedures will be evaluated after the patients are informed about their CHIP status at our hematology outpatient service via a questionnaire (Table S1). As fluctuations in QoL could potentially influence patient's satisfaction, completion of EORTC-QLQ-C30[83] and EORTC-QLQ-BR23[84] questionnaires will be requested in parallel. This allows a comparison with other cohorts of breast cancer patients[85] in case of unexpected results.
2.3.4 Cytokine profiling
Cytokines that are of interest in cardiometabolics are measured by custom-plex cytokine arrays (Eve Technologies) pre-treatment (Table 2). Measures will be performed in duplicates. These measurements will only be performed if > 15% of participants are CHIP-positive as a lower percentage would not allow for comparison between the CHIP-positive and -negative patients.
2.3.5 Quality control and missing data
Anonymized data will be entered in a database that complies with good clinical practice guidelines (Maganamed, https://maganamed.com/de). As patients might receive their therapy and routine blood work during therapy outside our center, missing data are expected. Thus, the rate of complete documentation is a part of the feasibility measures.
2.4 Sample size
The primary endpoint, defined as the estimation of willingness to participate with an accuracy of +/- 7.5%, is dependent on the real participation rate (p) and the number of approached patients (N). Tight confidence intervals (CIs) are associated with a superior accuracy of estimation and the width of the CI broadens with increased number of approached patients. In case of a real participation rate (p) of ~50% (p = 0.5), this estimation is the lowest and 200 patients would be required to achieve an accuracy of estimation of ±7.5%. The lower the real participation rate, the higher is the probability of a selection bias to characterize our cohort. That could potentially hamper the design of a future trial which aims at CHIP-tailored survivorship care in a real-world patient cohort. As an example: If the real participation rate is ≥ 80% which is expected, 80-100 patients would be in the range to estimate with the aimed accuracy. Table S3 summarizes the size of CIs in dependency of p and N. Based on case numbers at our center and the probability to include ~10-20% of patients who are CHIP-positive, we aim at a sample size of 80-100 patients to achieve in addition a sufficient power for assessment of feasibility metrics.
2.5 Statistical analysis
The primary analysis will assess the estimation of willingness to participate with an accuracy of +/- 7.5%. This estimation of willingness will be calculated with the Clopper-Pearson interval for the parameter p of the binominal distribution. For that, the limits of the confidence interval (95% CI(P)) represent the largest and the smallest, respectively, value of P that allows the assumption to achieve the estimated number of participants.
Secondary aims are to assess the patient acceptance of the study procedures and addition of CHIP diagnostics to the routine work-up, to evaluate the overall prevalence of pre-therapeutic CHIP in this cohort of breast cancer patients, to assess the feasibility to recruit 80-100 patients within 12 months, to assess the frequency of guideline-adherent cardiovascular preventive medications at baseline and after 12 months, and to characterize the prevalence of cardiovascular risk factors in CHIP-positive versus CHIP-negative patients.
3. Discussion
A growing body of evidence suggests that CHIP plays an important role for the development of TMN and cardio-metabolic toxicities besides its well-known impact on overall CV mortality. As early breast cancer has an excellent long-term survival, improvement of survivorship care is an unmet need. CHIP likely represents an important key factor for individualized, risk-tailored survivorship care. To the author's knowledge, this is the first study that prospectively implements CHIP diagnostics into routine clinical care of older adults with breast cancer. One major reason why the integration into routine clinical paths was neglected so far might be the lack of evidence how CHIP-positivity should be managed during survivorship care. Our pilot study will not answer this question but prepare further trials that focus on such topics. Thus, feasibility of recruitment and patient acceptance are our major outcomes to legitimate future trials or modify their design. Most survivorship programs are focussed on younger adults. As CHIP is related to aging, it provided the unique perspective to develop a tailored survivorship concept for older adults and their specific needs.
Most cancer patients have regularly scheduled visits with their cancer doctors (gynecologists, oncologists, hematologists). Thus, consultations with primary care specialists for purpose of prevention are often neglected. In line, cancer patients less often receive indicated preventive care assessments[86], cardiovascular medication[87], and counselling[88]. In concordance, a recent Canadian survey among hematologists revealed that 20% of them do not regularly consider the indication before they stop cardiovascular medication in acute myeloid leukemia patients and up to 28% did not recommence this medication after interruption[87]. Another survey described a discordance in perceived responsibilities for general preventive health between oncologists and general care physicians involved in survivorship care[89]. Similar data for a German cohort of cancer patients are lacking. To further elucidate this topic in our patient cohort, we will assess whether indicated preventive cardiovascular medication is prescribed at baseline and after 12 months. Although our cohort has a limited size, we expect an excellent quality of data in comparison to retrospective registry data as patients are scheduled for an outpatient visit that included a detailed history. If our assumption would be confirmed that breast cancer patients receive fewer preventive measures than indicated, this would provide a rationale to implement a close interaction between gynacologists, oncologists, and primary care physicians in our area.
4. Limitations
Several limitations should be addressed. First, trial results can be limited by the small sample size. If fewer than 15-20% of participants are CHIP-positive, this can result in a less informative result on patient satisfaction. In addition, serum cytokine analysis will not be performed in such a case. This would limit the significance of a sample size calculation, in order to prepare for the subsequent trial. As cardiovascular prevention is mainly initiated and supervised by primary care physicians, a further limitation is that their involvement is not intended so far. This could be compensated by a separate subsequent evaluation of general care physician's attitudes towards cardiovascular prevention in breast cancer patients based on the results of our study to address potential barriers.
We belief that the evaluation of patient satisfaction is a key strength of this study as the assessment and integration of patient's needs are a key requirement for future trial considerations. Another strength of this study are the broad inclusion criteria to avoid any relevant selection bias. This is especially important as prevalence of CV risk factors in breast cancer survivors was shown to be dependent on age-[88] and socioeconomic factors[90]. Although the recruiting center is a tertiary care hospital and academic institution, it serves as the main provider for breast cancer care in our region. Thus, no selection bias against older or unfit patients is expected. In addition, we expect an excellent retention rate at 12 months, as these visits are coupled with routine appointments.
5. Conclusions
The results of this study will inform us about the feasibility to integrate CHIP diagnostics into routine care of older breast cancer patients. If the primary endpoint is met and patient satisfaction is sufficient, patient characteristics of this cohort will allow us to design a large real-world study to assess a CHIP-tailored survivorship concept in order to improve treatment-related outcomes in this vulnerable patient group.
Supplementary materials
The supplementary material for this article is available at: Supplementary materials.
Authors contribution
Neuendorff NR: Writing-original draft, conceptualization, data curation, approval of final article.
Bittner AK, Hoffmann O, von Tresckow B, Kimmig R, Reinhardt HC, von Neuhoff N, Schneider M, Schmitz F: Conceptualization, approval of final article.
Mauer T: Writing-original draft, approval of final article.
Flossdorf S: Statistical conception, manuscript writing, approval of final article.
Köster J, Mölder F: Conceptualization, writing-original draft, approval of final article.
Turki AT: Clinical expertise, correction of manuscript, approval of final article.
Conflicts of interest
von Tresckow B reports grants or contracts from Novartis, Takeda, and Merck Sharp & Dohme; consulting fees from Allogene, BMS/Celgene, Cerus, Incyte, Mitlenyi, Novartis, Pentixapharm, Roche, Amgen, Pfizer, Takeda, Merck Sharp & Dohme, and Gilead Kite; honoraria from AstraZeneca, Novartis, Roche Pharma AG, Takeda, and Merck Sharp & Dohme; and support for attending meetings and/or travel from AbbVie, AstraZeneca, Kite-Gilead, Merck Sharp & Dohme, Takeda, and Novartis.
Neuendorff NR has received honoraria and travel support by Janssen-Cilag, Medac, Novartis, Pfizer, Abbvie, and Jazz Pharmaceutical.
Reinhardt HC reports research grants from the Deutsche Forschungsgemeinschaft, the Deutsche Krebshilfe, The State of Northrhine-Westfalia, the Bundesministerium für Bildung und Forschung, the Else Kröner-Fresenius Stiftung and the MERCUR Stiftung. Additional research grants were obtained from AstraZeneca and Gilead; consulting fees from BMS/Celgene, Novartis, Roche, Gilead; honoraria from AstraZeneca, Novartis, Roche AbbVie, Kite-Gilead, Merck Sharp & Dohme, Takeda, and Novartis. Reinhardt HC is a co-founder of CDL Therapeutics GmbH.
Turki AT: Consultancy for CSL Behring, Maat Pharma, Biomarin and Onkowissen.
Neuendorff NR is an Editorial Board member of Ageing and Cancer Research & Treatment.
All other authors have no conflicts of interest to declare.
Ethical approval
The protocol was approved by the Institutional Ethics Committee, University Duisburg-Essen on May 18, 2022.
Consent to participate
Written informed consents were obtained from all participants.
Consent for publication
Not applicable.
Availability of data and material
The data and materials could be obtained from the corresponding author.
Funding
This work is supported by University Duisburg-Essen, Medical Faculty, "Winterseminar reloaded (WIR)"-Grant to NRN, BVT, AKB, OH, and by Deutsche Forschungsgemeinschaft (DFG) in the framework of the DFG University Medicine Essen Clinician Scientist Academy (UMEA), FU 356/12-2 to NRN.
Copyright
© The Author(s) 2023.
References
-
1. Robert Koch-Institut, Gesellschaft der epidemiologischen Krebsregister in Deutschland e.V.. Krebs in Deutschland 2015/2016 [Internet]. Germany: Robert Koch-Institut. 2019. Available from: https://edoc.rki.de/handle/176904/6012
-
2. Janssen-Heijnen ML, van Steenbergen LN, Voogd AC, Tjan-Heijnen VCG, Nijhuis PH, Poortmans PM, et al. Small but significant excess mortality compared with the general population for long-term survivors of breast cancer in the Netherlands. Ann Oncol. 2014;25(1):64-68.
[DOI] -
3. Steensma DP, Bejar R, Jaiswal S, Lindsley RC, Sekeres MA, Hasserjian RP, et al. Clonal hematopoiesis of indeterminate potential and its distinction from myelodysplastic syndromes. Blood. 2015;126(1):9-16.
[DOI] -
4. Jaiswal S, Fontanillas P, Flannick J, Manning A, Grauman PV, Mar BG, et al. Age-related clonal hematopoiesis associated with adverse outcomes. N Engl J Med. 2014;371(26):2488-2498.
[DOI] -
5. Zink F, Stacey SN, Norddahl GL, Frigge ML, Magnusson OT, Jonsdottir I, et al. Clonal hematopoiesis, with and without candidate driver mutations, is common in the elderly. Blood. 2017;130(6):742-752.
[DOI] -
6. Coombs CC, Zehir A, Devlin SM, Kishtagari A, Syed A, Jonsson P, et al. Therapy-related clonal hematopoiesis in patients with non-hematologic cancers is common and associated with adverse clinical outcomes. Cell Stem Cell. 2017;21(3):374-382.
[DOI] -
7. Gillis N, Ball M, Zhang Q, Ma Z, Zhao Y, Yoder SJ, et al. Clonal haemopoiesis and therapy-related myeloid malignancies in elderly patients: a proof-of-concept, case-control study. Lancet Oncol. 2017;18(1):112-121.
[DOI] -
8. Takahashi K, Wang F, Kantarjian H, Doss D, Khanna K, Thompson E, et al. Preleukaemic clonal haemopoiesis and risk of therapy-related myeloid neoplasms: a case-control study. Lancet Oncol. 2017;18(1):100-111.
[DOI] -
9. Bolton KL, Ptashkin RN, Gao T, Braunstein L, Devlin SM, Kelly D, et al. Cancer therapy shapes the fitness landscape of clonal hematopoiesis. Nat Genet. 2020;52(11):1219-1226.
[DOI] -
10. Jaiswal S, Natarajan P, Silver AJ, Gibson CJ, Bick AG, Shvartz E, et al. Clonal hematopoiesis and risk of atherosclerotic cardiovascular disease. N Engl J Med. 2017;377(2):111-121.
[DOI] -
11. Khoury J, Solary E, Abla O, Akkari Y, Alaggio R, Apperley JF, et al. The 5th edition of the World Health Organization classification of haematolymphoid tumours: myeloid and histiocytic/dendritic neoplasms. Leukemia. 2022;36(7):1703-1719.
[DOI] -
12. Young AL, Challen GA, Birmann BM, Druley TE. Clonal haematopoiesis harbouring AML-associated mutations is ubiquitous in healthy adults. Nat Commun. 2016;7:12484.
[DOI] -
13. Desai P, Mencia-Trinchant N, Savenkov O, Simon MS, Cheang G, Lee S, et al. Somatic mutations precede acute myeloid leukemia years before diagnosis. Nat Med. 2018;24(7):1015-1023.
[DOI] -
14. Husby S, Favero F, Nielsen C, Sørensen BS, Bæch J, Grell K, et al. Clinical impact of clonal hematopoiesis in patients with lymphoma undergoing ASCT: a national population-based cohort study. Leukemia. 2020;34(12):3256-3268.
[DOI] -
15. Abelson S, Collord G, Ng SWK, Weissbrod O, Cohen NM, Niemeyer E, et al. Prediction of acute myeloid leukaemia risk in healthy individuals. Nature. 2018;559(7714):400-404.
[DOI] -
16. Spencer Chapman, Ranzoni AM, Myers B, Williams N, Coorens THH, Mitchell E, et al. Lineage tracing of human development through somatic mutations. Nature. 2021;595(7865):85-90.
[DOI] -
17. Mayerhofer C, Sedrak MS, Hopkins JO, Li T, Tayob N, Faggen MG, et al. Clonal hematopoiesis in older patients with breast cancer receiving chemotherapy. J Natl Cancer Inst. 2023;115(8):981-988.
[DOI] -
18. Fuster JJ, MacLauchlan S, Zuriaga MA, Polackal MN, Ostriker AC, Chakraborty R, et al. Clonal hematopoiesis associated with TET2 deficiency accelerates atherosclerosis development in mice. Science. 2017;355(6327):842-847.
[DOI] -
19. Cull AH, Snetsinger B, Buckstein R, Wells RA, Rauh MJ. Tet2 restrains inflammatory gene expression in macrophages. Exp Hematol. 2017;55:56-70.
[DOI] -
20. Zhang Q, Zhao K, Shen Q, Han Y, Gu Y, Li X, et al. Tet2 is required to resolve inflammation by recruiting Hdac2 to specifically repress IL-6. Nature. 2015;525(7569):389-393.
[DOI] -
21. Cook EK, Izukawa T, Young S, Rosen G, Jamali M, Zhang L, et al. Comorbid and inflammatory characteristics of genetic subtypes of clonal hematopoiesis. Blood Adv. 2019;3(16):2482-2486.
[DOI] -
22. Busque L, Sun M, Buscarlet M, Ayachi S, Feroz Zada, Provost S, et al. High-sensitivity C-reactive protein is associated with clonal hematopoiesis of indeterminate potential. Blood Adv. 2020;4(11):2430-2438.
[DOI] -
23. Cook EK, Izukawa T, Young S, Rosen G, Jamali M, Zhang L, et al. Comorbid and inflammatory characteristics of genetic subtypes of clonal hematopoiesis. Blood Adv. 2019;3(16):2482-2486.
[DOI] -
24. Wang S, Hu S, Luo X, Bao X, Li J, Liu M, et al. Prevalence and prognostic significance of DNMT3A-and TET2-clonal haematopoiesis-driver mutations in patients presenting with ST-segment elevation myocardial infarction. EBioMedicine. 2022;78:103964.
[DOI] -
25. Miller PG, Qiao D, Rojas-Quintero J, Honigberg MC, Sperling AS, Gibson CJ, et al. Association of clonal hematopoiesis with chronic obstructive pulmonary disease. Blood. 2022;139(3):357-368.
[DOI] -
26. Kuhnert S, Mansouri S, Rieger MA, Savai R, Avci E, Díaz-Piña G, et al. Association of clonal hematopoiesis of indeterminate potential with inflammatory gene expression in patients with COPD. Cells. 2022;11(13):2121.
[DOI] -
27. Wong WJ, Emdin C, Bick AG, Zekavat SM, Niroula A, Pirruccello JP, et al. Clonal hematopoiesis and risk of chronic liver disease. Nature. 2023;616(7958):747-754.
[DOI] -
28. Hecker JS, Hartmann L, Rivière J, Buck MC, van der Garde M, Rothenberg-Thurley M, et al. CHIP and hips: clonal hematopoiesis is common in patients undergoing hip arthroplasty and is associated with autoimmune disease. Blood. 2021;138(18):1727-1732.
[DOI] -
29. Bolton KL, Koh Y, Foote MB, Im H, Jee J, Sun CH, et al. Clonal hematopoiesis is associated with risk of severe Covid-19. Nat Commun. 2021;12(1):5975.
[DOI] -
30. Weber-Lassalle K, Ernst C, Reuss A, Möllenhoff K, Baumann K, Jackisch C, et al. Clonal hematopoiesis–associated gene mutations in a clinical cohort of 448 patients with ovarian cancer. J Natl Cancer Inst. 2022;114(4):565-570.
[DOI] -
31. Coffee B, Cox HC, Kidd J, Sizemore S, Brown K, Manley S, et al. Detection of somatic variants in peripheral blood lymphocytes using a next generation sequencing multigene pan cancer panel. Cancer Genet. 2017;211:5-8.
[DOI] -
32. Chang CM, Lin KC, Hsiao NE, Hong WA, Lin CY, Liu TC, et al. Clinical application of liquid biopsy in cancer patients. BMC cancer. 2022;22(1):413.
[DOI] -
33. Sun MY, Lin FQ, Chen LJ, Li H, Lin WQ, Du HY, et al. Targeted next-generation sequencing of circulating tumor DNA mutations among metastatic breast cancer patients. Curr Oncol. 2021;28(4):2326-2336.
[DOI] -
34. Severson EA, Riedlinger GM, Connelly CF, Vergilio JA, Goldfinger M, Ramkissoon S, et al. Detection of clonal hematopoiesis of indeterminate potential in clinical sequencing of solid tumor specimens. Blood. 2018;131(22):2501-2505.
[DOI] -
35. Feng Y, Newsome R, Robinson T, Bowman RL, Zuniga A, Hall K, et al. Dnmt3a mutations in the hematopoietic system promote colitis-associated colon cancer: A model of clonal hematopoiesis in solid tumors. Blood. 2021;138(Suppl 1):2161.
[DOI] -
36. Zhao P, Xia N, Zhang H, Deng T. The metabolic syndrome is a risk factor for breast cancer: a systematic review and meta-analysis. Obesity Facts. 2020;13(4):384-396.
[DOI] -
37. Dong S, Wang Z, Shen K, Chen X. Metabolic syndrome and breast cancer: prevalence, treatment response, and prognosis. Front Oncol. 2021;11:629666.
[DOI] -
38. Fuster JJ, Zuriaga MA, Zorita V, MacLauchlan S, PolackalMN , Viana-Huete V, et al. TET2-loss-of-function-driven clonal hematopoiesis exacerbates experimental insulin resistance in aging and obesity. Cell Rep. 2020;33(4):108362.
[DOI] -
39. Ortmann CA, Dorsheimer L, Abou-El-Ardat K, Hoffrichter J, Assmus B, Bonig H, et al. Functional dominance of CHIP-mutated hematopoietic stem cells in patients undergoing autologous transplantation. Cell Rep. 2019;27(7):2022-2028.
[DOI] -
40. Wolff AC, Blackford AL, Visvanathan K, Rugo HS, Moy B, Goldstein LJ, et al. Risk of marrow neoplasms after adjuvant breast cancer therapy: the national comprehensive cancer network experience. J Clin Oncol. 2015;33(4):340-348.
[DOI] -
41. Kida M, Usuki K, Uchida N, Fukuda T, Katayama Y, Kondo T, et al. Outcome and risk factors for therapy-related myeloid neoplasms treated with allogeneic stem cell transplantation in Japan. Biol Blood Marrow Transplant. 2020;26(8):1543-1551.
[DOI] -
42. Zekavat SM, Viana-Huete V, Jorshery SD, Zuriaga MA, Uddin MM, Trinder M, et al. TP53-mediated clonal hematopoiesis confers increased risk for incident peripheral artery disease. medRxiv [Preprint]. 2021.
[DOI] -
43. Bhattacharya R, Zekavat SM, Haessler J, Fornage M, Raffield L, Uddin MM, et al. Clonal hematopoiesis is associated with higher risk of stroke. Stroke. 2022;53(3):788-797.
[DOI] -
44. Arends CM, Liman TG, Strzelecka PM, Kufner A, Löwe P, Huo SF, et al. Associations of clonal hematopoiesis with recurrent vascular events and death in patients with incident ischemic stroke. Blood. 2023;141(7):787-799.
[DOI] -
45. Libby P. Interleukin-1 beta as a target for atherosclerosis therapy: biological basis of CANTOS and beyond. J Am Coll Cardiol. 2017;70(18):2278-2289.
[DOI] -
46. Svensson EC, Madar A, Campbell CD, He YS, Sultan M, Healey ML, et al. TET2-driven clonal hematopoiesis and response to canakinumab: an exploratory analysis of the CANTOS randomized clinical trial. JAMA Cardiol. 2022;7(5):521-528.
[DOI] -
47. Heyde A, Rohde D, McAlpine CS, Zhang S, Hoyer FF, Gerold JM, et al. Increased stem cell proliferation in atherosclerosis accelerates clonal hematopoiesis. Cell. 2021;184(5):1348-1361.
[DOI] -
48. Yu B, Roberts MB, Raffield LM, Zekavat SM, Nguyen NQH, Biggs ML, et al. Supplemental Association of clonal hematopoiesis with incident heart failure. J Am Coll Cardiol. 2021;78(1):42-52.
[DOI] -
49. Pascual-Figal DA, Bayes-Genis A, Díez-Díez M, Hernández-Vicente A, Vázquez-Andrés D, de la Barrera J, et al. Clonal hematopoiesis and risk of progression of heart failure with reduced left ventricular ejection fraction. J Am Coll Cardiol. 2021;77(14):1747-1759.
[DOI] -
50. Sano S, Oshima K, Wang Y, MacLauchlan S, Katanasaka Y, Sano M, et al. Tet2-mediated clonal hematopoiesis accelerates heart failure through a mechanism involving the IL-1β/NLRP3 inflammasome. J Am Coll Cardiol. 2018;71(8):875-886.
[DOI] -
51. Sano S, Wang Y, Yura Y, Sano M, K Oshima, Yang Y, et al. JAK2V617F-mediated clonal hematopoiesis accelerates pathological remodeling in murine heart failure. JACC Basic Transl Sci. 2019;4(6):684-697.
[DOI] -
52. Lanza O, Ferrera A, Reale S, Solfanelli G, Petrungaro M, Tini Melato G, et al. New insights on the toxicity on heart and vessels of breast cancer therapies. Med Sci. 2022;10(2):27.
[DOI] -
53. Zhang M, Yang H, Xu C, Jin F, Zheng A. Risk factors for anthracycline-induced cardiotoxicity in breast cancer treatment: a meta-analysis. Front Oncol. 2022;12:899782.
[DOI] -
54. Mitchell JD, Cehic DA, Morgia M, Bergom C, Toohey J, Guerrero PA, et al. Cardiovascular manifestations from therapeutic radiation: a multidisciplinary expert consensus statement from the International Cardio-Oncology Society. JACC CardioOncol. 2021;3(3):360-380.
[DOI] -
55. Szczepaniak P, Siedlinski M, Hodorowicz-Zaniewska D, Nosalski R, Mikolajczyk TP, Dobosz AM, et al. Breast cancer chemotherapy induces vascular dysfunction and hypertension through a NOX4-dependent mechanism. J Clin Invest. 2022;132(13):e149117.
[DOI] -
56. Kwan ML, Cheng RK, Iribarren C, Neugebauer R, Rana JS, Nguyen-Huynh M, et al. Risk of cardiometabolic risk factors in women with and without a history of breast cancer: the pathways heart study. J Clin Oncol. 2022;40(15):1635-1646.
[DOI] -
57. Battisti NML, Welch CA, Sweeting M, de Belder M, Deanfield J, Weston C, et al. Prevalence of cardiovascular disease in patients with potentially curable malignancies: a national registry dataset analysis. JACC CardioOncol. 2022;4(2):238-253.
[DOI] -
58. Hatakeyama K, Hieda M, Semba Y, Moriyama S, Wang Y, Maeda T, et al. TET2 clonal hematopoiesis is associated with anthracycline-induced cardiotoxicity in patients with lymphoma. JACC CardioOncol. 2022;4(1):141-143.
[DOI] -
59. Calvillo-Argüelles O, Schoffel A, Capo-Chichi JM, Abdel-Qadir H, Schuh A, Carrillo-Estrada M, et al. Cardiovascular disease among patients with AML and CHIP-related mutations. JACC CardioOncol. 2022;4(1):38-49.
[DOI] -
60. Sano S, Wang Y, Ogawa H, Horitani K, Sano M, Polizio AH, et al. TP53-mediated therapy-related clonal hematopoiesis contributes to doxorubicin-induced cardiomyopathy by augmenting a neutrophil-mediated cytotoxic response. JCI Insight. 2021;6(13):e146076.
[DOI] -
61. Afifi AM, Saad AM, Al-Husseini MJ, Elmehrath AO, Northfelt DW, Sonbol MB, et al. Causes of death after breast cancer diagnosis: A US population-based analysis. Cancer. 2020;126(7):1559-1567.
[DOI] -
62. Bradshaw PT, Stevens J, Khankari N, Teitelbaum SL, Neugut AI, Gammon MD. Cardiovascular disease mortality among breast cancer survivors. Epidemiology. 2016;27(1):6-13.
[DOI] -
63. Patnaik JL, Byers T, DiGuiseppi C, Dabelea D, Denberg TD. Cardiovascular disease competes with breast cancer as the leading cause of death for older females diagnosed with breast cancer: a retrospective cohort study. Breast Cancer Res 2011;13:R61.
[DOI] -
64. Ramin C, Schaeffer ML, Zheng Z, Connor AE, Hoffman-Bolton J, Lau B, et al. All-cause and cardiovascular disease mortality among breast cancer survivors in CLUE II, a long-standing community-based cohort. J Natl Cancer Inst. 2021;113(2):137-145.
[DOI] -
65. Zhang X, Pawlikowski M, Olivo-Marston S, Williams KP, Bower JW, Felix AS. Ten-year cardiovascular risk among cancer survivors: the National Health and Nutrition Examination Survey. PloS One. 2021;16(3):e0247919.
[DOI] -
66. Strongman H, Gadd S, Matthews AA, Mansfield KE, Stanway S, Lyon AR, et al. Does cardiovascular mortality overtake cancer mortality during cancer survivorship? An English retrospective cohort study. JACC CardioOncol. 2022;4(1):113-123.
[DOI] -
67. Armenian SH, Xu L, Ky B, Sun C, Farol LT, Pal SK, et al. Cardiovascular disease among survivors of adult-onset cancer: a community-based retrospective cohort study. J Clin Oncol. 2016;34(10):1122-1130.
[DOI] -
68. Chow EJ, Chen Y, Hudson MM, Feijen EAM, Kremer LC, Border WL, et al. Prediction of ischemic heart disease and stroke in survivors of childhood cancer. J Clin Oncol. 2018;36(1):44-52.
[DOI] -
69. Law W, Johnson C, Rushton M, Dent S. The Framingham risk score underestimates the risk of cardiovascular events in the HER2-positive breast cancer population. Curr Oncol. 2017;24(5):e348-e353.
[DOI] -
70. Fredslund SO, Gravholt CH, Laursen BE, Jensen AB. Key metabolic parameters change significantly in early breast cancer survivors: an explorative PILOT study. J Transl Med. 2019;17(1):105.
[DOI] -
71. Oliveri S, Ferrari F, Manfrinati A, Pravettoni G. A systematic review of the psychological implications of genetic testing: a comparative analysis among cardiovascular, neurodegenerative and cancer diseases. Front Genet. 2018;9:624.
[DOI] -
72. Chavarri-Guerra Y, Slavin TP, Longoria-Lozano O, Weitzel JN. Genetic cancer predisposition syndromes among older adults. J Geriatr Oncol. 2020;11(7):1054-1060.
[DOI] -
73. Sella T, Fell GG, Miller PG, Gibson CJ, Rosenberg SM, Snow C, et al. Patient perspectives on testing for clonal hematopoiesis of indeterminate potential. Blood Adv. 2022;6(24):6151-6161.
[DOI] -
74. Neuendorff NR, Frenzel LP, Leuschner F, Fremd C. Integrating clonal haematopoiesis into geriatric oncology: The ARCH between aging, cardiovascular disease and malignancy. J Geriatr Oncol. 2021;12(3):479-482.
[DOI] -
75. Fossa S. Quality-of-life assessment in unselected oncologic out-patients-a pilot-study. Int J Oncol. 1994;4(6):1393-1397.
[DOI] -
76. SCORE2 working, ESC Cardiovascular. SCORE2 risk prediction algorithms: new models to estimate 10-year risk of cardiovascular disease in Europe. Eur Heart J. 2021;42(25):2439-2454.
[DOI] -
77. Mölder F, Jablonski KP, Letcher B, Hall MB, Tomkins-Tinch CH, Sochat V, et al. Sustainable data analysis with Snakemake. F1000Res. 2021;10:33.
[DOI] -
78. Li H. Aligning sequence reads, clone sequences and assembly contigs with BWA-MEM. arXiv:1303.3997 [Preprint]. 2013.
[DOI] -
79. DePristo MA, Banks E, Poplin R, Garimella KV, Maguire JR, Hartl C, et al. A framework for variation discovery and genotyping using next-generation DNA sequencing data. Nat Genet. 2011,43(5):491-498;
[DOI] -
80. Köster J, Dijkstra LJ, Marschall T, Schönhuth A. Varlociraptor: enhancing sensitivity and controlling false discovery rate in somatic indel discovery. Genome Biol. 2020;21(1):98.
[DOI] -
81. McLaren W, Gil L, Hunt SE, Riat HS, Ritchie GRS, Thormann A, et al. The ensembl variant effect predictor. Genome Biol. 2016;17(1):122.
[DOI] -
82. Hartmann T, Schröder C, Kuthe E, Lähnemann D, Köster J, et al. Insane in the vembrane: filtering and transforming VCF/BCF files. Bioinformatics. 2023;39(1):btac810.
[DOI] -
83. Aaronson NK, Ahmedzai S, Bergman B, Bullinger M, Cull A, Duez NJ, et al. The European Organization for Research and Treatment of Cancer QLQ-C30: a quality-of-life instrument for use in international clinical trials in oncology. J Natl Cancer Inst. 1993;85(5):365-376.
[DOI] -
84. Sprangers MA, Groenvold M, Arraras JI, Franklin J, te Velde A, Muller M, et al. The European Organization for Research and Treatment of Cancer breast cancer-specific quality-of-life questionnaire module: first results from a three-country field study. J Clin Oncol. 1996;14(10):2756-2768.
[DOI] -
85. Cheng KK, Lim EY, Kanesvaran R. Quality of life of elderly patients with solid tumours undergoing adjuvant cancer therapy: a systematic review. BMJ Open. 2018;8(1):e018101.
[DOI] -
86. Snyder CF, Frick KD, Kantsiper ME, Peairs KS, Herbert RJ, Blackford AL, et al. Prevention, screening, and surveillance care for breast cancer survivors compared with controls: changes from 1998 to 2002. J Clin Oncol. 2009;27(7):1054-1061.
[DOI] -
87. Durand M, Lacaria K, Sidsworth M, Davis MK, Sanford D. Management of cardiovascular health in acute leukemia: a national survey. Leuk Lymphoma. 2019;60(12):2982-2992.
[DOI] -
88. Enright KA, Krzyzanowska MK. Control of cardiovascular risk factors among adult cancer survivors: a population-based survey. Cancer Cause Control. 2010;21(11):1867-1874.
[DOI] -
89. Cheung WY, Neville BA, Cameron DB, Cook EF, Earle CC. Comparisons of patient and physician expectations for cancer survivorship care. J Clin Oncol. 2009;27(15):2489-2495.
[DOI] -
90. Thotamgari SR, Sheth AR, Grewal US. Racial disparities in cardiovascular disease among patients with cancer in the United States: The Elephant in the Room. eClinicalMedicine. 2022;44:101297.
[DOI]
Copyright
© The Author(s). This is an Open Access article licensed under a Creative Commons Attribution 4.0 International License (https://creativecommons.org/licenses/by/4.0/), which permits unrestricted use, sharing, adaptation, distribution and reproduction in any medium or format, for any purpose, even commercially, as long as you give appropriate credit to the original author(s) and the source, provide a link to the Creative Commons license, and indicate if changes were made.
Publisher’s Note
Share And Cite