- 1 Curtin Medical School, Curtin Health Innovation Research Institute, Faculty of Health Sciences, Curtin University, WA 6102, Australia.
Abstract
Western and third world countries alike are experiencing population ageing with people living longer. The World Health Organization website states that ‘between 2015 and 2050, the proportion of the world's population over 60 years will nearly double from 12% to 22% reaching 2.1 billion’, and that ‘the number of persons aged 80 years or older is expected to triple between 2020 and 2050 to reach 426 million’. However, the elderly (i.e., those aged over 65 years) are 11 times more likely to develop cancer than younger people; this is illustrated by GLOBOCAN 2020 data showing that greater than 50% of people who had cancer were 65 or older in 2018. This age-related cancer emergence may in part be due to increasing dysregulation of the immune system or “immunosenescence”. Macrophages are pivotal immune cells in maintaining homeostasis and in regulating inflammatory responses during immunological insults, such as cancer, where they can perform anti-tumourigenic functions. Yet, tumour-associated macrophages are well known for their ability to promote tumour growth, with numbers often correlating to cancer progression and poorer outcomes. Macrophages contribute to this by secreting growth and angiogenic factors, and they closely interact with endothelial cells and cancer cells to help shape the tumour microenvironment. During ageing, macrophage response to environmental stimuli becomes dysregulated including impaired anti-tumour functions. Furthermore, increased number of macrophages and precursor cells are observed in lymphoid organs that can supply to tumours with ageing. Such age-related changes, including those to endothelial cells, may promote cancer development and lead to poorer cancer outcomes in elderly people. In this review, we discuss recent findings concerning how macrophages are modulated during healthy ageing and in cancer, with a focus on macrophage and endothelial cell interactions.
Keywords
1. Ageing, Cancer and Inflamm-ageing
Western and third world countries alike are experiencing population ageing with people living longer. The number of people aged 60 years and over is predicted to reach 2.1 billion by 2050[1]. However, ageing is a risk factor for many chronic diseases, such as diabetes, dementia, cardiovascular diseases and cancer. Notably, the elderly (i.e., those aged over 65 years) are 11 times more likely to develop cancer than younger people; this is illustrated by GLOBOCAN 2020 data showing that greater than 50% of people who had cancer were 65 or older in 2018[2]. This may be due to several biological changes associated with ageing, including inflamm-ageing[3] and immunosenescence[4]. Inflamm-ageing describes low-grade, chronic inflammation that can occur during ageing in the absence of infection[3,5]. This is characterised by an imbalance of pro- and anti-inflammatory factors. For example, increased circulating levels of interleukin (IL)-1β[6,7], IL-6[7-14], IL-18[6,11,13], tumour-necrosis factor (TNF)[7,8,12,15-17], IL-10[7,13] and transforming growth factor (TGF)-β[6,18,19]. This low but sustained inflammatory state may promote the pathogenesis of age-associated diseases, such as cancer.
2. Immunosenescence and Cancer
The immune system can control cancer development and growth however immune function changes during ageing, known as immunosenescence[4]. It is well established that T and B cell function is altered with age. For example, within the T cell compartment, naïve cells decrease and memory cells increase with age[20-23]. Additionally, a decline in peripheral B cell numbers and diversity occurs with ageing[24-26]. Innate immunity is also impacted with ageing, where monocytes and macrophages may exist in a higher state of basal activation[27,28] and produce more pro-inflammatory mediators such as TNF-α[29] and IL-6[30,31]. However, their response to stimulation is also impaired leading to poorer responses to infection[31,32]. Chronic activation of the innate immune system during ageing, including macrophages, contributes to inflamm-ageing[3]. Overall, inflamm-ageing and immunosenescence can impact immune cell function, which will inevitably affect the outcome of cancer.
3. Macrophage Overview
Macrophages constitute a heterogeneous immune cell population that are pivotal in maintaining homeostasis by performing a range of tasks including development, tissue repair, phagocytosis, and regulating inflammatory responses (reviewed in[33]). It is therefore not surprising that macrophages exhibit plasticity and can quicky adapt to their local microenvironment to perform specialised functions. Tissue resident macrophages (TRMs) are long-lived cells that occupy all organs and tissues of the body, playing an important role in regulating local tissue-specific immune responses (reviewed in[34]). Even within an organ, different macrophage populations can exhibit functional differences and perform unique tasks[35-37]. For example, marginal zone macrophages in the spleen are adept at phagocytosing pathogens present in the circulation. Whereas splenic red pulp macrophages are uniquely positioned and specialised at clearing red blood cells and debris from circulation and are important for iron homeostasis[36]. Interestingly, the spleen can harbour a large reservoir of monocytes and macrophages which can be deployed as a compensatory response, secondary to the bone marrow (BM)[38-40], during severe injury, infection or cancer.
4. Macrophage Ontogeny
Ontogenically, TRMs can be derived during embryonic development (yolk sac and foetal liver), seed different tissues, and are maintained throughout life[37,41-44]. Conversely, BM haematopoietic stem cells (HSC) can generate macrophages through a monocyte intermediate (monocyte-derived macrophages; MDM), migrate to tissues and adopt a specific transcriptional program to resemble TRMs (reviewed in[37]). These ontogenically distinct macrophages may have different and overlapping features. Embryonic macrophages appear to have a higher proliferation and self-maintenance capacity compared to BM-derived macrophages (BMMs)[45-47]. Single-cell RNA-sequencing revealed yolk sac-derived arterial macrophages were enriched in vascular endothelial growth factor (VEGF) production, tissue regeneration, chemotaxis, and apoptosis in response to vascular inflammation[47]. Whereas BMMs were enriched in biological pathways involving inflammatory responses, cytokine secretion, leukocyte aggregation and chemotaxis[47]. In contrast, van de Laar et al.[46] showed that once yolk sac, foetal liver-derived macrophages and BMMs colonised the alveolar space, they developed into long-lived, self-maintaining alveolar macrophages, with a nearly indistinguishable gene expression profile. Additionally, these cells were functionally equivalent in their ability to clear surfactant[46]. Thus, the function of ontogenically distinct TRMs may be similar or different depending on the tissue they colonise[48] and if they are under homeostatic or inflammatory conditions.
5. Macrophage Function
Macrophages display functional plasticity depending on the microenvironment they inhabit and can exhibit a spectrum of inflammatory phenotypes (Figure 1). Pro-inflammatory factors, such as lipopolysaccharides (LPS) and interferon-gamma (IFN-γ), can lead to inflammatory macrophage function characterised by inducible nitric oxide synthase (iNOS), produce reactive oxygen species (i.e., nitric oxide), IFN-γ, IL-6, IL-1β, IL-12, TNF-α, C-C motif chemokine ligand (CCL)2 (also known as monocyte chemoattractant protein-1; MCP-1) and CCL5 (also known as regulated upon activation, normal T cell expressed and secreted; RANTES)[49,50]. These mediators recruit immune cells such as inflammatory monocytes, macrophages and T cells, and support T helper (Th)1 activation. Macrophages can also exhibit anti-inflammatory functions required for wound healing via increased expression of the mannose receptor CD206, arginase 1 (Arg1), and prostaglandin E2 (PGE2)[51], along with secretion of IL-4, IL-5, IL-10, VEGF, TGF - β , CCL17 (also known as thymus- and activation-regulated chemokine; TARC) and CCL22 (also known as macrophage-derived cytokine; MDC)[49,50,52]. These factors lead to the recruitment of CC chemokine receptor (CCR)4+ regulatory T cells (Tregs) and other lymphocytes and drive a Th2 response. Various stimuli can activate anti-inflammatory macrophages such as IL-4, IL-13, immune complexes, IL-10 and via phagocytosis of apoptotic cells[49,52], with variations in cytokine and chemokine expression profiles. During muscle injury/tissue repair, pro-inflammatory macrophages may switch to exhibit wound healing macrophage characteristics required for repair[53,54]. Pulmonary macrophages can exhibit both pro and anti-inflammatory markers, such as CD11c, CD206, and Ym1 (also known as chitinase-like protein 3; Chil3)[35,55]. Additionally, anti-tumourigenic macrophages may initially infiltrate the tumour microenvironment only to be subverted to a pro-tumourigenic or mixed phenotype[56,57]. Thus, macrophage adoption of a functional phenotype is dynamic and driven by microenvironmental changes[58].
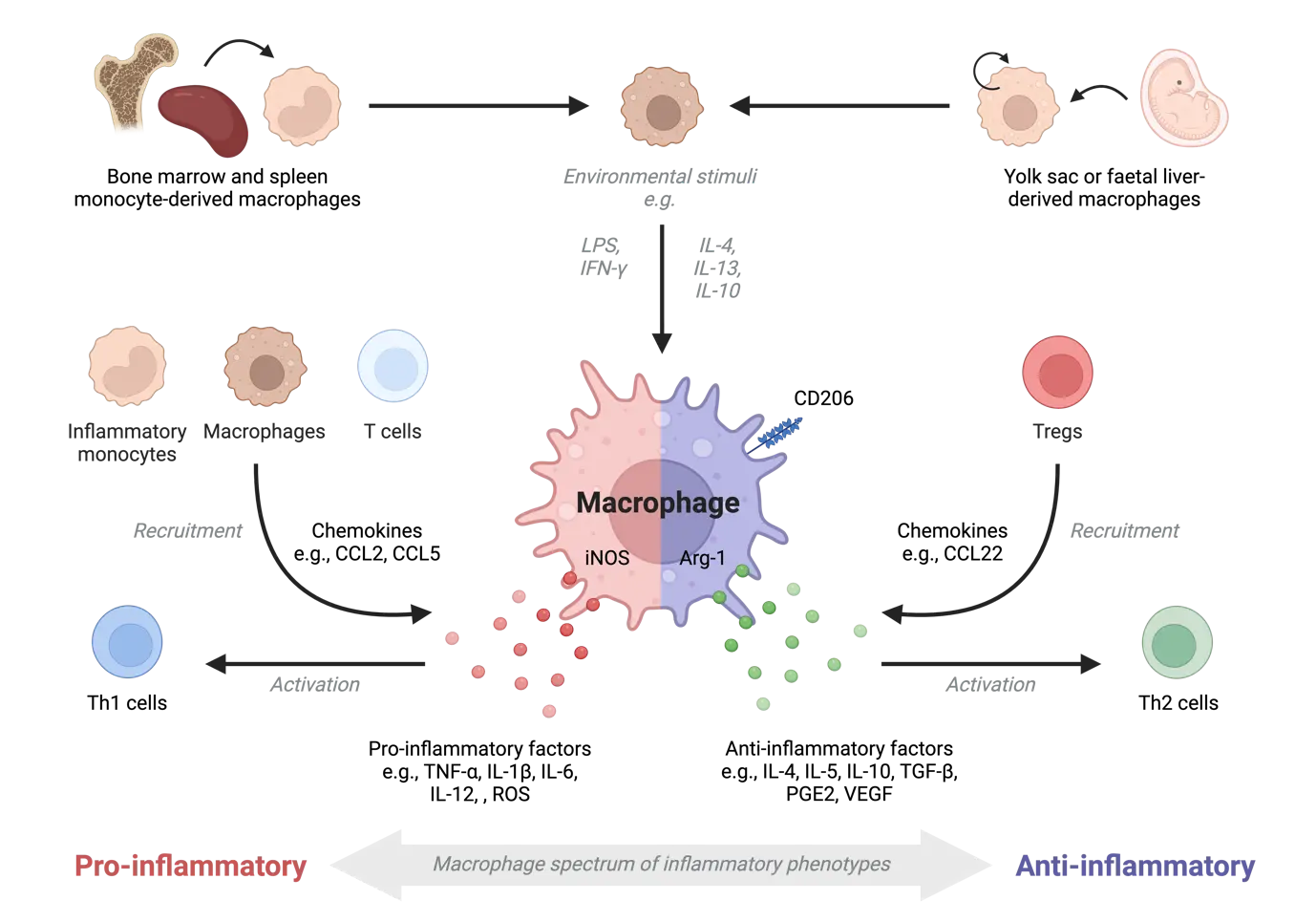
Figure 1. Macrophage origin and inflammatory function. Macrophages can be derived from the bone marrow/spleen via a monocyte intermediate or established during embryonic development (yolk sac and foetal liver). Macrophages can exhibit pro-inflammatory and anti-inflammatory functions when exposed to stimuli, such as LPS/IFN-γ or IL-4/IL-13, respectively. Pro-inflammatory macrophages can secrete chemokines to activate Th1 cells and recruit other leukocytes, such as inflammatory monocytes, macrophages and T cells. Anti-inflammatory macrophages can recruit Tregs and activate Th2 cells. Macrophages are highly plastic and can exhibit both pro- and anti-inflammatory functions. Created in BioRender.com. LPS: lipopolysaccharides; IFN-γ: interferon-gamma; IL: interleukin; CCL: chemokine ligand; Th: T helper; TGF: transforming growth factor; PGE2: prostaglandin E2; VEGF: vascular endothelial growth factor; iNOS: inducible nitric oxide synthase; Tregs: regulatory T cells.
6. Macrophage-Endothelial Cell Interactions
Interactions between macrophages and endothelial cells (ECs) are critical for inflammatory responses and regulation of vascular function. ECs can express chemotactic factors that recruit monocytes and macrophages, such as colony stimulating factor (CSF)-1 (also known as macrophage colony stimulating factor; M-CSF)[59] and CCL2[60,61] during microvascular growth and remodelling[62]. ECs can then contribute to monocyte to macrophage differentiation and activation[59,61,63-65], in turn macrophages closely interact with blood vessels to coordinate angiogenesis[52,66,67] (reviewed in[68]).
Monocyte to macrophage conversion begins in the vasculature during inflammation and is mediated by CCR2 signalling, resulting in the generation of pro-inflammatory immature macrophages[61]. ECs can then mediate conversion of macrophages towards an anti-inflammatory phenotype, as shown with BMMs co-cultured with ECs in vitro[59,65]. This is mediated through endothelial angiocrine factors that instruct macrophage programming. For example, EC-specific production of lactate activated macrophages towards an anti-inflammatory phenotype that was essential for muscle revascularisation and regeneration after ischemia in mice[64]. Additionally, interstitial macrophages responded to lung EC-derived Rspondin3, a Wnt signalling modulator, by increasing anti-inflammatory IL-10, Arg1 and CD206 expression, and downregulating the pro-inflammatory markers TNF and iNOS[65]. Interestingly, Willenborg et al.[69] showed infiltrating inflammatory CCR2+ monocytes differentiated into macrophages that highly expressed VEGF to promote tissue vascularisation in skin wounds in mice. These VEGF-expressing macrophages predominated at early stages of tissue repair and displayed a mixed inflammatory phenotype, expressing IL-6, iNOS and Arg1. Whereas late stages of repair involved mainly anti-inflammatory macrophages[69]. Overall, these studies show the dynamic nature of macrophage activation program which can be modulated by ECs to promote angiogenesis and wound repair.
The involvement of different macrophage subsets in angiogenesis has been delineated by several studies in vitro and in vivo. Pro-inflammatory macrophages were found at the leading front of sprouts[52] and secreted TNF-α and VEGF to promote vascular sprouting by inducing EC tip formation[70,71]. Furthermore, vessel length, number and branch points increased in the presence of pro-inflammatory macrophages[52]. However, these pro-angiogenic effects were only observed in the short-term (one day after macrophage co-culture with ECs), as longer exposure was associated with vessel regression[52]. Anti-inflammatory macrophages were seen wrapped around vessels and bridged neighbouring sprouts[66]. This included involvement in anastomosis (fusion of new blood vessels), promotion of vascular remodelling through production of matrix metallopeptidases (MMPs), including MMP-9[52,71], and upregulation of TGFb-1 which is involved in vessel maturation, as well as EC migration and proliferation[51,52].
The studies above mostly describe macrophages derived from BM, yet macrophage origin and ontogeny may also impact their angiogenic function. Yolk-sac-derived TRMs were found to be superior at promoting angiogenesis in the brain compared to BMMs[66]. Similarly, pro-angiogenic and wound healing pathways were enriched in kidney TRMs versus BMMs[72]. This was further demonstrated in vitro where kidney TRMs increased transcriptional expression of VEGF-A, IL-10, ARG-1, TGFb-2 and promoted EC proliferation[72]. Thus, a shift in macrophage ontogeny with ageing, from embryonic-derived TRMs to BMM, may impact on macrophage-EC interaction and vascular function.
7. Macrophages and Healthy Ageing
Macrophages undergo numerical and functional changes with healthy ageing, which varies depending on tissue site (Table 1). Dermal[73], alveolar[74-76] and peritoneal[77] macrophages were shown to be reduced in aged mice compared to young mice. In contrast, visceral adipose tissue macrophages in mice[78] and those in human skeletal muscle[79-82] remain unchanged with ageing. It is, however, well documented that monocytes and/or macrophages increase in the BM[83-86], spleen[84,85] and liver[87,88] with ageing in mice and rats. Interestingly, these are key organs involved in haematopoiesis (referred to as extramedullary haemopoiesis in the spleen and liver[38,39] (reviewed in[89]), suggesting that more monocytes and macrophages are available for deployment in the elderly, if necessary. Limited human data exists for BM and spleen, although studies on human circulating monocytes indicate they are elevated[90,91] or remain similar to their younger counterparts with ageing[92]. There appears to be a consensus that a bias towards myelopoiesis over lymphopoiesis occurs with ageing[93-96]. This suggests an age-related increase in the number of myeloid progenitors, monocytes and macrophages that occupy hematopoietic organs which supply peripheral tissues during inflammation.
Age-related changes | Species/strain | Sex | Ref |
Numerical | |||
↓ Dermal (skin) | C57BL/6J mice | Female | [73] |
↓ Alveolar | C57BL/6 miceC57BL/6 and BALB/c | Both Not specified | [74-76] |
↓ Peritoneal | C57BL/6 mice | Not specified | [77] |
= Visceral adipose tissue | C57BL/6 mice | Male | [78] |
= Skeletal muscle | HumansBALB/c | Both Not specified | [79-82] |
↑ Bone marrow | C57BL/6 mice | Both | [83-86] |
↑ Spleen | C57BL/6J mice | Female | [84,85] |
↑ Liver | Fischer 344 rats | Male | [87,88] |
↑ Blood monocytes | Humans | Both | [90,91] |
↑ Myelopoiesis/Lymphopoiesis | C57BL/6 miceC57BL/6, DBA/2 miceHumans | Both Not specifiedBoth | [93-96] |
Phenotype and functional (in vivo or directly ex vivo) | |||
↑ IL-10+ macrophages in bone marrow and spleen | C57BL/6J mice | Female | [85] |
↑ IL-10 production in peritoneal macrophages | C57BL/6 mice | Male | [97] |
↓ CD206 ↑ IL-1β ↑ MHC-II+ bone marrow macrophages | C57BL/6J mice | Male | [99] |
↑ TNF-α circulating monocytes | Humans | Not specified | [100] |
↑ Anti-inflammatory macrophages in skeletal muscle | HumansBALB/c, C57BL/6 mice | Both Not specified | [82,98] |
→ Pro-inflammatory macrophages in adipose tissue | C57BL/6 mice | Male | [78] |
→ Pro-inflammatory macrophages in gastrointestinal tract | C57BL/6 mice | Male | [101] |
↑ Pro- and anti-inflammatory markers in alveolar macrophages | C57BL/6 mice | Female | [74] |
↑ Activation markers (CD80, CD86) and ↑ alveolar macrophage numbers in LPS-induced acute lung injury model | C57BL/6J mice | Male | [102] |
Hyperinflammatory dermal macrophages during wound healing | C57BL/6J mice | Female | [73] |
Functional (in vitro) | |||
↓ IL-6 and TNF-ɑ in splenic and peritoneal macrophages stimulated with LPS and S. aureus | C57BL/6 mice | Female | [103] |
↓ TNF-ɑ in LPS-stimulated peritoneal macrophages | BALB/c mice | Female | [104] |
↓ IL-6 and TNF-ɑ in blood monocytes stimulated with Pam3CSK4 and polyuridylate | Humans | Both | [105] |
↓ IL-6 and TNF-ɑ in blood monocytes stimulated with Pam3CSK4 | Humans | Not specified | [106] |
↓ IL-6, IL-12, IL-1β, TNF-ɑ and ↑IL-10 in LPS-stimulated splenic macrophages | BALB/c mice | Not specified | [107] |
↓ IL-6, IL-1β, TNF-ɑ in alveolar macrophages stimulated with S pneumoniae | BALB/c mice | Female | [32] |
↑ IL-6 ↑ TNF in LPS-stimulated murine BMDMs and human PBMC-derived macrophages | C57BL/6 miceHumans | Female Not specified | [30] |
↑ IL-6 in unstimulated and LPS-stimulated BMMs | C57BL/6 mice | Female | [31] |
↑ PGE2 in LPS-stimulated peritoneal macrophages | C57BL/6NIA mice | Male | [108] |
↓ MHC class II in IFN𝛾-stimulated BMDMs | C57BL/6 mice | Not specified | [109] |
↓ IL-6 in unstimulated BMDMs; ↓ IL-6 ↑ CCL2 in IL-4-stimulated BMDMs; ↓ IL-6 ↑ TNF in IFN𝛾-stimulated BMDMs | C57BL/6 mice | Male | [110] |
Phagocytosis | |||
↓ Apoptotic cells Jurkat cells and senescent neutrophils by BMMs | C57BL/6 mice | Male | [86,99] |
↓ Apoptotic neutrophils by alveolar macrophages | C57BL/6 mice | Not specified | [75] |
↓ Fluorescent latex beads by splenic macrophages | C57BL/6J mice | Female | [111] |
↓ Fluorescent particles by peritoneal macrophages | C57BL/6 mice | Male | [97] |
↓ Radiolabelled mitochondria by Kupffer cells | Sprague-Dawley rats | Female | [112] |
↓ Neutrophils by Kupffer cells | BM/BiRij rats | Female | [113] |
↓ pHRODO-labelled E. coli by blood monocytes | Humans | Both | [29] |
Ontogeny | |||
Embryonically derived microglia maintained; Yolk sac-derived arterial macrophage diminished in elderly, whilst BMMs increased until middle age and remained stable with ageing | RankCre Rosa26eYFP (C57BL/6J background) | Not specified | [47] |
Microglia predominantly embryonic-derivedBladder TRMs slowly maintained by BMMs | Flt3Cre Rosa26YFP (C57BL/6 background) | Male | [120] |
Alveolar TRMs maintained with ageing but is depleted and replaced by BMMs during sublethal exposure to influenza A virus | C57BL/6J mice | Male | [76] |
Skin TRMs replaced by MDMs with ageing | Humans | Female | [122] |
Ovarian TRMs replaced by MDMs with ageing | C57BL/6J mice | Female | [123] |
MDMs transplanted into adult mice replaced 80-90% of cardiac TRMs within 8 weeks (to at least 36 weeks) | C57BL/6 mice | Not specified | [124] |
↑: increased; ↓: decreased; →: shift towards; =: no changes; MDM: monocyte-derived macrophages; TRM: tissue resident macrophages; BMM: bone marrow-derived macrophages; LPS: lipopolysaccharides; PGE2: prostaglandin E2; IL: interleukin; TNF: tumour-necrosis factor; MHC: major histocompatibility complex.
The BM and spleens of elderly mice were reported to harbour increased anti-inflammatory IL-10+ macrophages compared to young mice[85] and isolated peritoneal macrophages produced more IL-10 with ageing[97]. Similarly, skeletal muscle in humans and mice have increased anti-inflammatory macrophages that were associated with increased fibrosis and collagen accumulation[82,98]. Moreover, this was linked to aged BM contribution to the muscle[98]. In contrast, BMMs isolated from elderly mice increased pro-inflammatory IL-1β expression[99] and circulating monocytes from elderly humans produced more TNF-α and MCP-1[100]. Furthermore, macrophages in adipose tissues[78] and the gastrointestinal tract[101] shift to a pro-inflammatory phenotype with ageing. At steady state, lung macrophages from aged mice exhibited upregulation of both pro- and anti-inflammatory markers, such as nitric oxide synthase 2 (NOS-2), CCL8 (also known as monocyte chemoattractant protein-2; MCP-2), chemokine (C-X-C motif) ligand 9 (CXCL9), Arg1 and TGFβ-3, compared to younger mice[74]. Yet, their ability to appropriately respond to infection or injury is compromised. For example, lung macrophages in the elderly were hyporesponsive, with diminished IL-6, IL-1β and TNF-α production during infection with Streptococcus pneumoniae[32]. In contrast, in response to acute lung injury, lung macrophages in the elderly were hyperresponsive, with enhanced macrophages numbers and activation, likely contributing to poorer outcomes in the elderly[102]. In a different context, dermal macrophages in elderly mice were also found to be hyperinflammatory during wound healing, with increased expression of IL-6, IL-1β, CCL2, nucleotide oligomerisation domain (NOD-)like receptor family pyrin domain containing 3 (NLRP3), hypoxia inducible factor 1 subunit alpha (HIF-1α), TNF and nuclear factor kappa B subunit 1 (NFκB-1)[73]. Furthermore, cell cycle, DNA repair and replication pathways were downregulated in elderly relative to young macrophages[73]. These age-related changes were concomitant with increased tissue damage[73].
Further evidence of age-related dysregulation in macrophage responses to stimuli have been demonstrated in in vitro studies. Murine macrophages and human monocytes exhibited reduced expression of the pro-inflammatory cytokines IL-6 and TNF-α when stimulated with TLR ligands, including LPS and Staphylococcus aureus[103-106]. Chelvarajan et al.[107] also demonstrated that macrophages in response to LPS decreased secretion of the pro-inflammatory factors IL-1β, IL-6, TNF-α and IL-12, but increased expression of anti-inflammatory IL-10. Additionally, LPS-stimulated macrophages in the elderly increased production of PGE2, which implies increased suppressive function, as PGE2 upregulates IL-10, but reduces IL-12 production and major histocompatibility complex (MHC) class II expression[108]. MHC class II expression in IFN-γ-stimulated BMMs was markedly reduced in elderly versus young mice, suggesting reduced antigen presentation to CD4+ T cells[109]. In contrast, macrophages in the elderly may have an enhanced ability to mobilise other monocytes and macrophages, as they upregulated CCL2 expression when stimulated with IL-4[110].
Generally, macrophages have reduced phagocytic ability with ageing, shown in BMMs[86,99], splenic[111], alveolar[75], peritoneal macrophages[97] and Kupffer cells[112,113] in rodents, and in human blood monocytes[29]. Phagocytosis is important for resolution of inflammation[114,115], thus, age-related impairment of phagocytosis in macrophages can lead to accumulation of damaged and senescent cells. The latter has been shown to accumulate with age[116-118], likely disrupting tissue homeostasis and contributing to chronic inflammation in the elderly.
Shifts in ontogenically distinct TRMs with ageing vary depending on the tissue site. Liu et al. demonstrated that monocytes contributed to the pool of TRMs over time and at different rates (comparison of mice up to 9 months of age), where the gut and dermis occurred the fastest, followed by the kidney, spleen and peritoneal cavity, which had a slower contribution from monocytes[119]. In contrast, the liver, brain, and epidermis remained predominantly of embryonic origin[119]. Studies investigating macrophage ontogeny in elderly mice showed that brain microglia maintain their embryonic origin[47], whilst bladder TRMs were slowly replaced by BMMs, yet a considerable proportion of embryonic-derived cells persisted with ageing[120]. Yolk sac-derived arterial TRMs were the predominant population until adulthood, with absolute numbers plateauing in 10-month-old mice and then diminishing with advanced age[47]. Furthermore, BM-derived arterial macrophages increased to similar numbers as their yolk sac counterparts at 10 months, yet their numbers remained steady until at least 20-21 months of age[47]. In contrast, alveolar TRMs were maintained over the lifespan of mice at steady state[76] but could be depleted and replaced by BMMs during severe injury, such as from bleomycin-induced fibrosis or sublethal exposure to influenza A virus[76,121]. Furthermore, these BMMs persisted for at least 10 months after injury in the lungs[121]. Overall, this suggests that the TRM landscape in terms of ontogeny may be impacted by tissue type, age and previous inflammatory encounters. The shift in macrophage ontogeny may impact tissue homeostasis and macrophage response to inflammation and repair.
8. Tumour-Associated Macrophages
Macrophages are implicated in many cancers, including mesothelioma and lung cancer[56,84,125-128], where they can make up to half of the total tumour burden[125,129-131]. Indeed, tumour-associated macrophage (TAM) numbers are often associated with poorer outcome[132-134] due to their ability to promote tumour growth[59,125,135], invasion, metastasis[132,136], neoangiogenesis[51,59,71] and therapeutic resistance[137-139]. On the flip side, TAMs can be anti-tumourigenic, involved in destroying cancer cells, phagocytosis, activating tumour antigen-specific effector T cells[140], and mediating antibody dependent cellular cytotoxicity and phagocytosis (ADCC and ADCP, respectively)[141-143].
Chronic inflammation serves as the foundation of neoplastic development[144] in which TRMs, present in the tissue prior to the carcinogenic event, are amongst the first to engage with the transformed cells and are important in shaping the tumour microenvironment[135]. TRMs can be hijacked to support tumour growth[145] through production of growth and angiogenic factors[146]. Tumour cells and stroma can secrete chemotactic factors, such as CCL2, CCL5, CSF-1 and VEGF that can recruit monocytes and macrophages to the tumour[147-152]. Initially, monocyte-derived TAMs may be pro-inflammatory and anti-tumourigenic. However, tumour-derived factors, such as IL-4, IL-10 and TGF-β, can promote functional reprogramming towards anti-inflammatory and pro-tumour functions[152,153]. Indeed, elevated immune suppressive TAMs are often associated with tumour progression and poor prognosis[126,127,130,154-157]. This phenotypic switch may be incomplete, as TAMs can display a mixed pro- and anti-inflammatory phenotype (i.e., IL-10 and TNF-α), as shown in human pancreatic ductal adenocarcinoma[158] and mouse mesothelioma tumours[56].
TAMs can exert a dual effect on the efficacy of cancer treatments, including immune checkpoint inhibitors (ICI). For example, the efficacy of anti-cytotoxic T-lymphocyte associated protein (CTLA)-4 treatment on murine mesenchymal-like glioblastoma tumour growth was reliant on microglial TAM-mediated activation of CD4+ tumour infiltrating T cells via MHC class II[159]. In turn, IFN-γ produced by CD4+ T cells upregulated microglial TAM phagocytosis and tumour cell removal[159]. In metastatic melanoma, patients that responded to anti-CTLA-4 therapy had reduced tumour-infiltrating Tregs, and increased CD68+ TAMs and circulating monocytes that expressed CD16 (also known as FcγRIIIA)[160]. Interestingly, the latter was shown to eradicate Tregs bound with anti-CTLA-4 antibodies via ADCC-mediated lysis ex vivo. In contrast, TAMs hampered the efficacy of anti-programmed death (PD)-1 therapy by protecting PD-1+ tumour infiltrating CD8+ T cells[137]. This involved the transfer of the bound anti-PD-1 monoclonal antibodies on T cells to TAMs via Fcγ receptors, in murine melanoma, and lung and colon adenocarcinoma models[137]. Distinct TAM phenotypes may contribute to the differences in response to immunotherapy. For example, using single cell RNA-seq, Rashidian et al.[161] showed that suppressive TAMs (with increased CD206 and Arg-1 expression) accumulated in murine colorectal adenocarcinoma tumours of non-responders to anti-PD-1 blockade, concomitant with reduced infiltration of CD8+ T cells. Additionally, Peronzoni et al.[162] demonstrated that CD206+ stromal TAMs were responsible for excluding CD8+ T cell infiltration into lung squamous cell carcinoma tumour islets and elimination of TAMs with anti-CSF-1 receptor (CSF-1R) antibodies improved anti-PD-1 treatment efficacy in young mice.
TAMs of distinctive origin contribute differently to tumour development. This has been elegantly demonstrated by Casanova-Acebes et al.[135] showing TRMs accumulate near non-small cell lung cancer cells after inoculation in mice. However, with tumour progression, monocyte-derived TAM numbers and proportions increased. TRMs and MDMs were functionally distinct, wherein the former was shown to promote tumour remodelling, tumour cell migration and invasiveness, while the latter upregulated DNA replication and cell-cycle progression responses in tumour cells[135]. In a murine model of glioblastoma, Antunes et al. 2021[163] showed microglia-derived TAMs were functionally distinct to monocyte-derived TAMs. Microglia-derived TAMs exhibited increased secretion of the pro-inflammatory factors TNF, CCL2 and CCL4 (also known as macrophage inflammatory protein-1 beta; MIP-1β), which suggests an enhanced ability to recruit microglia, monocytes/macrophages, and T cells to the tumour. However, microglia-derived TAMs strongly suppressed T cell proliferation. Both TAM populations have enhanced but comparable pro-angiogenic activity however, monocyte-derived TAMs outcompeted microglial-derived TAMs in hypoxic regions of the murine and human glioblastoma tumours, suggesting they have an important role to play in tumour angiogenesis[163]. In a murine lung cancer model, tissue-resident TAMs were also found to support the growth of tumour cells and promote angiogenesis[164], while monocyte-derived TAMs facilitated tumour remodelling and spreading in lungs. Furthermore, monocyte-derived TAMs accumulated in the tumour with cancer progression and became the predominant TAM population[164].
9. Tumour-Associated Macrophages and Tumour Endothelial Cells in Tumour Angiogenesis
During disease progression oxygen-deprived or hypoxic regions emerge in solid tumours. This is because in the early stages of development, tumour cells rely on diffusion of oxygen and nutrients from surrounding tissue. If tumour cells continue to proliferate, the local environment becomes hypoxic. Hypoxia increases expression of transcription factors or coactivators in tumour cells, ECs and macrophages, such as hypoxia-inducible factor-1 (HIF-1) and peroxisome proliferator activated receptor gamma (PPARγ) coactivator (PGC)-1α that induce production of angiogenic factors, in particular VEGF and angiopoietin (Ang)2[165,166]. Hypoxia is also a potent inducer of chemokines such as CCL2, CCL5, as well as factors that directly influence monocytes and macrophages such as CSF-1 and IL-6 by tumour cells, ECs and TAMs[155,167], which attract additional TAMs to accumulate in these areas. These TAMs further promote neoangiogenesis via upregulation of angiogenic factors, including IL-6[168,169], VEGF[168], MMP-9[136,170] and TNF-α (reviewed in Henze[171]).
ECs can directly drive pro-tumourigenic functions in macrophages whilst they traverse blood vessels and engage with ECs. For example, macrophages cultured with ECs and injected back into mice were activated towards an anti-inflammatory, pro-angiogenic phenotype and resulted in increased angiogenesis and faster prostate tumour growth[59]. Activation of TAMs towards this state may be facilitated by tumour endothelial cell (TEC)-derived factors, such as IL-6, which was shown to be necessary for the activation of suppressive IL-10+ TAMs, as EC-specific knockout of IL-6 reduced the number of these TAMs and improved anti-cancer outcomes in a model of glioblastoma tumour in young mice[155]. A complementary study showed that EC-derived Ang2 (which is upregulated by IL-6 signalling)[166,168] promoted activation of pro-angiogenic monocytes/macrophages that expressed Tie2 (receptor for Ang2). Furthermore, overexpression of Ang2 in ECs led to increased infiltration of Tie2+ TAMs into Lewis Lung carcinoma (LLC) tumours which resulted in increased microvessel formation, however at the cost of EC maturity, contributing to the tumours becoming more hypoxic[172]. Tie2 and Ang2 have been shown to be critical for TAM and EC interaction as blocking either of these molecules abrogated the ability of TAMs to closely associated with blood vessels to promote angiogenesis and subsequently resulted in reduced tumour growth and metastasis in a murine breast cancer model[173].
10. Tumour-Associated Macrophages and Ageing
The expansion of myeloid precursors, monocytes and macrophages in the BM with ageing suggests the elderly have a greater potential to supply these cells in response to tumour-derived factors. We have shown that there is a larger pool of splenic monocytes and macrophages with healthy ageing (non-tumour-bearing hosts) and this was further exaggerated with mesothelioma tumour progression in mice[84]. This corresponded with an expansion of TAMs and tumour growth in elderly mice[84,125]. Similarly, TAMs increased with ageing in murine prostate cancer[174] and colorectal cancer in humans and mice[175]. Whereas TAMs in human oral squamous cell carcinoma were found to be similar between young and elderly patients[176]. Regardless, immune suppressive TAMs are associated with cancer progression (as discussed previously) and predominate in tumours of elderly patients (prostate cancer[131]) and mice (mesothelioma and prostate cancer[84,131]).
Functionally, aged macrophages appear to have impaired anti-tumour responses with decreased cytotoxicity towards cancer cells[177] alongside a reduced ability to activate T cells[178], both shown in vitro. We have shown that TAMs in elderly mesothelioma-bearing mice impaired anti-tumour T cell function and contributed to poorer response to IL-2/anti-CD40 immunotherapy, compared to complete tumour regression in young mice[125]. Furthermore, macrophage depletion improved responses to IL-2/CD40 treatment in elderly but not young mice[125]. This suggests TAMs in the elderly are more suppressive and contribute to poorer anti-cancer outcomes (Table 2 and Figure 2). The impact of macrophages on the efficacy of other immunotherapies in the elderly is yet to be determined.
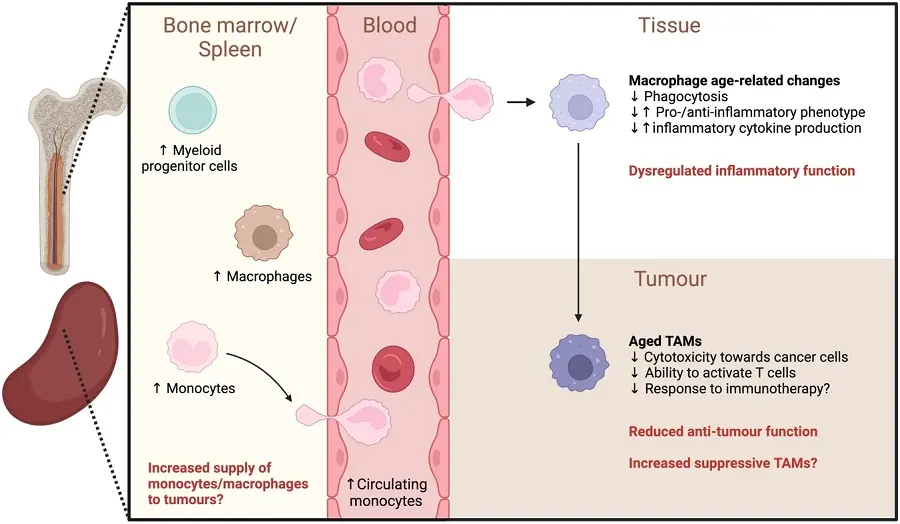
Figure 2. Age-related changes that may impact tumour-associated macrophages. Elderly hosts have the potential to supply more monocytes/macrophages to tumours due to an increase in myeloid progenitor cells, monocytes and macrophages in the bone marrow and spleen with ageing. Aged macrophages exhibit dysregulated function, including reduced phagocytic ability and altered inflammatory cytokine production in response to stimuli. The inflammatory phenotype of macrophages in different tissues are also altered with age. Aged monocytes/macrophages can give rise to TAMs with reduced anti-tumour activity (decreased cytotoxicity and ability to activate T cells) and may contribute to poorer response to immunotherapy in the elderly. Suppressive TAMs may also increase with ageing. Created in BioRender.com. ↑: increased; ↓: decreased; TAMs: tumour-associated macrophages.
Age-related changes | Species/strain | Sex | Ref |
Numerical | |||
↑ Mesothelioma tumour, corresponding to faster tumour growth | C57BL/6J mice | Female | [125] |
↑ Prostate cancer | C57BL/6 mice | Male | [174] |
↑ Colorectal cancer | BALB/c miceHumans | Not specified Not specified | [175] |
= Oral squamous cell carcinoma | Humans | Both | [176] |
Phenotype and functional | |||
↑ Immune suppressive TAMs in mesothelioma | C57BL/6 mice | Female | [84] |
↑ Immune suppressive TAMs in prostate cancer | Humans (TCGA dataset)C57BL/6 mice | Male | [131] |
↓ Anti-tumour T cell function and contributes to poor response to IL2/anti-CD40 immunotherapy (improved with macrophage depletion in elderly but not young mice) | C57BL/6J mice | Female | [125] |
↓ Anti-tumour response with ↓ cytotoxicity towards cancer cells in peritoneal macrophages (in vitro) | C57BL/6 mice | Both | [177] |
↓ Ability to activate T cells (in vitro) | C57BL/6 mice | Female | [178] |
↑: increased; ↓: decreased; =: no changes; TCGA: the cancer genome atlas; TAMs: tumour-associated macrophages.
11. Tumour-Associated Macrophages, Tumour Endothelial Cells and Ageing
Whilst it is well known that ECs change with the ageing process, also known as vascular ageing, the effect of ageing on TECs and how they influence the tumour microenvironment is less well understood. There is evidence that ECs from aged mice show decreased proliferation and migration[179-181] contributing to delayed wound healing. Moreover, aged individuals appear to have impaired physiological angiogenesis with ECs adopting a senescent profile (reviewed in[182]). Yet, even in elderly hosts, TECs proliferate to drive angiogenesis and promote tumour growth, albeit in a dysregulated manner. It is possible that the aged tumour environment contributes to TEC dysfunction because accumulating senescent ECs in vessels, such as those seen in the aortic wall of aged mammals, have been shown to induce EC dysfunction, along with increased permeability[181,183-186]. Structural and functional changes in senescent ECs include vascular leak, thrombosis and immune dysregulation, key hallmarks of cancer. Furthermore, senescent ECs exhibit increased expression and secretion of various cytokines, such as IL-6 and CCL2[181,187], known as the senescence-associated secretory phenotype (SASP)[188]. SASP cytokines cause low-grade chronic inflammation, cellular fibrosis, and promote macrophage infiltration which collectively exacerbate vascular ageing[181]. However, ECs can also express a non-activated, potentially anti-inflammatory senescent population, which is induced by age-related stress[189] and by overexpression of the vascular protective gene ARHGAP18 (also called SENEX)[190]. This anti-inflammatory senescent phenotype is mediated through caveolae and inhibition of NFκB[191]. The proportion of pro-inflammatory and anti-inflammatory senescent ECs and TECs in aged hosts may determine the fate of tumorigenesis in premalignant cells.
Macrophages recruited by SASP factors can clear senescent cell, such as TECs and tumour cells[192]. However, macrophage phagocytosis is impeded with ageing and inadequate removal of these senescent cells may lead to continual inflammation in support of cancer development and growth. Indeed, a study by Haston et al.[193] demonstrated that removal of senescent cells, which predominantly consisted of TAMs (mainly with a pro-tumoural phenotype) followed by TECs, resulted in reduced Kirsten rat sarcoma virus (KRAS)-driven lung tumour growth in young mice. In effect, the tumour vasculature was reduced, along with increased CD8+ T cells and reduced FoxP3+ Tregs in the tumours. Furthermore, administration of anti-CSF-1R antibody depleted senescent TAMs (while TECs were unaffected) which led to reduced tumour burden, demonstrating the direct involvement of senescent TAMs in cancer progression[193]. The same study also established that macrophages and ECs similarly made up the majority of the senescent cell population in the lungs of healthy elderly mice[193]. However, further studies are required to directly link the role of age-associated senescent macrophages and ECs in tumour initiation and progression in the elderly.
As discussed earlier, pro-tumoural TAMs are important in tumour angiogenesis and appear to accumulate in many tumours of elderly humans and mice. This suggests that angiogenesis in the ageing tumour may be enhanced, likely in a dysregulated manner, by the increased presence of these pro-angiogenic TAMs. Studies in this area are currently lacking, however one study showed that immune suppressive TAMs expanded in intraocular tumours in mature (10-12-month-old) but not young (2-month-old) mice[194]. Furthermore, depletion of these cells in the eye resulted in downregulation of genes associated with angiogenesis, such as VEGF, Tie2 and CD31, concomitant with reduced tumour growth in the older mice[194]. Although this study did not assess whether TAMs directly interacted with TECs to promote angiogenesis, it does suggest that ageing may drive TAMs towards pro-angiogenic functions and promote tumour growth in older hosts. Whether this is due to age-related cell intrinsic factors or as a result of the instructive niche provided by aged TECs[59] is yet to be determined. More studies are required to address how ageing modulates TAM and TEC interaction and the impact on cancer progression in the elderly.
Another question remains as to whether the changes to the proportion of ontogenically distinct TAMs can impact tumour angiogenesis and progression. As discussed earlier, healthy ageing is associated with the gradual replacement of embryonic-derived TRMs with BMMs in certain organs. Although some studies in young mice demonstrated that TAMs of different ontogeny have distinct functions, including angiogenesis, it is yet to be determined how age-related changes to these cells, in relation to numbers and function, can directly impact cancer progression in elderly.
12. Summary and Conclusions
Macrophages play a significant role in health and disease, such as in cancer, where they can contribute to poor outcomes due to their relative abundance and pro-tumourigenic functions. One important aspect is the crosstalk between macrophages and ECs that help drive pro-tumourigenic functions in macrophages, including promoting tumour angiogenesis. Ageing can modulate macrophages in several ways, including increased numbers of monocytes/macrophages and myeloid precursor cells that supply tumours, dysregulating responses to stimuli, and inducing functional changes, such as decreased phagocytosis and impaired anti-tumour responses. Thus, age-related changes to macrophages may contribute to poorer cancer outcomes in the elderly, however few studies have addressed this. A better understanding of how ageing modulates TAMs, along with their interaction with TECs, to drive tumour angiogenesis and progression is required Figure 3 illustrates the potential interaction of TAMs and TECs during ageing that may impact cancer outcomes in the elderly. This may lead to the development of new therapeutic strategies to improve anti-cancer outcomes in elderly people.
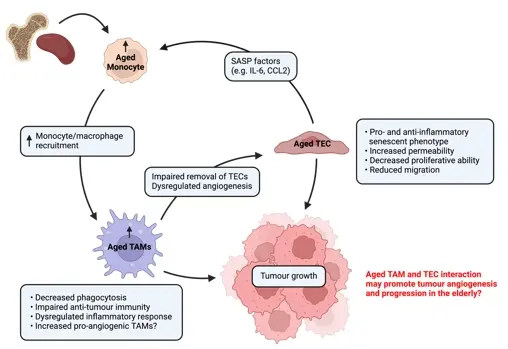
Figure 3. Age-related changes to tumour-associated macrophages and their interaction with tumour endothelial cells that may contribute to cancer progression in the elderly. Aged TECs secrete SASP factors, such as IL-6 and CCL2, which can signal to monocytes and macrophages in the bone marrow and spleen, resulting in their mobilisation and recruitment to the tumour where they interact with TECs. An age-related expansion of myeloid progenitors and monocytes/macrophages suggests increased potential to supply to tumours. TAMs in the elderly have reduced anti-tumour function, dysregulated inflammatory response and reduced phagocytic ability, which mean they are unable to effectively remove senescent/aged TECs. Additionally, there may be increased pro-angiogenic TAMs in tumours of the elderly. The interaction of TAMs and TECs may promote tumour angiogenesis and progression in the elderly. Created in Biorender.com. SASP: senescence-associated secretory phenotype; TEC: tumour endothelial cell; TAM: tumour-associated macrophage; CCL: chemokine ligand; IL: interleukin.
Acknowledgements
Duong L was supported by a PhD top up scholarship from Cancer Council Western Australia.
Authors contribution
All authors contributed equally to this work.
Conflicts of interest
Nelson DJ is a research academic at Curtin University and is involved in a number of research projects. One project is funded by an immunotherapy start-up company, Selvax. Selvax played no role in the preparation, or generation of data described in this manuscript.
Nelson DJ is an Editorial Board member of Ageing and Cancer Research & Treatment.
Ethical approval
Not applicable.
Consent to participate
Not applicable.
Consent for publication
Not applicable.
Availability of data and materials
Not applicable.
Funding
None.
Copyright
© The Author(s) 2024.
References
-
1. World Health Organization. Ageing and health [Internet]. 2022. Available from: https://www.knowledge-action-portal.com/en/content/ageing-and-health
-
2. Data visualization tools for exploring the global cancer burden in 2022 [Internet]. France: International Agency for Research on Cancer, 2020 [cited 2024 Jan 22]. Available from: https://gco.iarc.fr/today
-
3. Franceschi C, Bonafè M, Valensin S, Olivieri F, De Luca M, Ottaviani E, et al. Inflamm-aging. An evolutionary perspective on immunosenescence. Ann NY Acad Sci. 2000;908:244-254.
[DOI] -
4. Fulop T, Kotb R, Fortin CF, Pawelec G, De Angelis, Larbi A. Potential role of immunosenescence in cancer development. Ann NY Acad Sci. 2010;1197(1):158-165.
[DOI] -
5. Franceschi C, Garagnani P, Parini P, Giuliani C, Santoro A. Inflammaging: a new immune–metabolic viewpoint for age-related diseases. Nat Rev Endocrinol. 2018;14(10):576-590.
[DOI] -
6. Niu XH, Xie YP, Yang S, Chen Y, Xu L, Zhang Y, et al. IL-18/IL-18R1 promotes circulating fibrocyte differentiation in the aging population. Inflamm Res. 2020;69(5):497-507.
[DOI] -
7. Rodríguez L, López-Hoyos M, Muñoz-Cacho P, Martínez-Taboada VM. Aging is associated with circulating cytokine dysregulation. Cell Immunol. 2012;273(2):124-132.
[DOI] -
8. Parker D, Sloane R, Pieper CF, Hall KS, Kraus VB, Kraus WE, et al. Age-related adverse inflammatory and metabolic changes begin early in adulthood. J Gerontol A Biol Sci Med Sci. 2019;74(3):283-289.
[DOI] -
9. Wei J, Xu H, Davies JL, Hemmings GP. Increase of plasma IL-6 concentration with age in healthy subjects. Life Sci. 1992;51(25):1953-1956.
[DOI] -
10. Hager K, Machein U, Krieger S, Platt D, Seefried G, Bauer J. Interleukin-6 and selected plasma proteins in healthy persons of different ages. Neurobiol Aging. 1994;15(6):771-772.
[DOI] -
11. Ferrucci L, Corsi A, Lauretani F, Bandinelli S, Bartali B, Taub DD, et al. The origins of age-related proinflammatory state. Blood. 2005;105(6):2294-2299.
[DOI] -
12. Valiathan R, Ashman M, Asthana D. Effects of Ageing on the Immune System: Infants to Elderly. Scand J Immunol. 2016;83(4):255-266.
[DOI] -
13. Morrisette-Thomas V, Cohen A, Fülöp T, Riesco É, Legault V, Li Q, et al. Inflamm-aging does not simply reflect increases in pro-inflammatory markers. Mech Ageing Dev. 2014;139:49-57.
[DOI] -
14. Stowe RP, Peek MK, Cutchin MP, Goodwin JS. Plasma Cytokine Levels in a Population-Based Study: Relation to Age and Ethnicity. J Gerontol A Biol Sci Med Sci. 2010;65(4):429-433.
[DOI] -
15. Franceschi C, Campisi J. Chronic inflammation (inflammaging) and its potential contribution to age-associated diseases. J Gerontol A Biol Sci Med Sci. 2014;69(Suppl 1):S4-S9.
[DOI] -
16. Bruunsgaard H, Skinhøj P, Pedersen A, et al. Ageing, tumour necrosis factor-alpha (TNF-alpha) and atherosclerosis. Clin Exp Immunol. 2000;121(2):255-260.
[DOI] -
17. Davizon-Castillo P, McMahon B, Aguila S, Schroll M, Pedersen BK. TNF-α–driven inflammation and mitochondrial dysfunction define the platelet hyperreactivity of aging. Blood. 2019;134(9):727-740.
[DOI] -
18. Carrieri G, Marzi E, Olivieri F, Marchegiani F, Cavallone F, Cardelli M, et al. The G/C915 polymorphism of transforming growth factor β1 is associated with human longevity: a study in Italian centenarians. Aging Cell. 2004;3(6):443-448.
[DOI] -
19. Forsey RJ, Thompson JM, Ernerudh J, Hurst TL, Strindhall J, Johansson B, et al. Plasma cytokine profiles in elderly humans. Mech Ageing Dev. 2003;124(4):487-493.
[DOI] -
20. Fagnoni FF, Vescovini R, Passeri G, Bologna G, Pedrazzoni M, Lavagetto G, et al. Shortage of circulating naive CD8+ T cells provides new insights on immunodeficiency in aging. Blood. 2000;95(9):2860-2868.
[DOI] -
21. Li M, Yao D, Zeng X, Kasakovski D, Zhang Y, Chen S, et al. Age related human T cell subset evolution and senescence. Immun Ageing. 2019;16(1):24-24.
[DOI] -
22. Czesnikiewicz-Guzik M, Lee W-W, Cui D, Hiruma Y, Lamar DL, Yang ZZ, et al. T cell subset-specific susceptibility to aging. Clin Immunol. 2008;127(1):107-118.
[DOI] -
23. Quinn KM, Fox A, Harland KL, Russ BE, Li J, Nguyen THO, et al. Age-Related Decline in Primary CD8+ T Cell Responses Is Associated with the Development of Senescence in Virtual Memory CD8+ T Cells. Cell Rep. 2018;23(12):3512-3524.
[DOI] -
24. Bulati M, Buffa S, Candore G, Caruso C, Dunn-Walters DK, Pellicano M, et al. B cells and immunosenescence: A focus on IgG+IgD-CD27- (DN) B cells in aged humans. Ageing Res Rev. 2011;10(2):274-284.
[DOI] -
25. Chong Y, Ikematsu H, Yamaji K, Nishimura M, Nabeshima S, Kashiwagi S, et al. CD27+ (memory) B cell decrease and apoptosis-resistant CD27- (naive) B cell increase in aged humans: implications for age-related peripheral B cell developmental disturbances. Int Immunol. 2005;17(4):383-390.
[DOI] -
26. Gibson KL, Wu YC, Barnett Y, Duggan O, Vaughan R, Kondeatis E, et al. B-cell diversity decreases in old age and is correlated with poor health status. Aging Cell. 2009;8(1):18-25.
[DOI] -
27. Smallwood HS, López-Ferrer D, Squier TC. Aging enhances the production of reactive oxygen species and bactericidal activity in peritoneal macrophages by upregulating classical activation pathways. Biochemistry. 2011;50(45):9911-9922.
[DOI] -
28. Barman PK, Shin JE, Lewis SA, Kang S, Wu D, Wang Y, et al. Production of MHCII-expressing classical monocytes increases during aging in mice and humans. Aging Cell. 2022;21(10):e13701-e13701.
[DOI] -
29. Hearps AC, Martin GE, Angelovich TA, Cheng WJ, Maisa A, Landay AL, et al. Aging is associated with chronic innate immune activation and dysregulation of monocyte phenotype and function. Aging Cell. 2012;11(5):867-875.
[DOI] -
30. Bouchlaka MN, Sckisel GD, Chen M, Mirsoian A, Zamora AE, Maverakis E, et al. Aging predisposes to acute inflammatory induced pathology after tumor immunotherapy. J Exp Med. 2013;210(11):2223-2237.
[DOI] -
31. Thevaranjan N, Puchta A, Schulz C, Naidoo A, Szamosi JC, Verschoor CP, et al. Age-Associated Microbial Dysbiosis Promotes Intestinal Permeability, Systemic Inflammation, and Macrophage Dysfunction. Cell Host Microbe. 2017;21(4):455-466.
[DOI] -
32. Boyd AR, Shivshankar P, Jiang S, Berton MT, Orihuela CJ. Age-related defects in TLR2 signaling diminish the cytokine response by alveolar macrophages during murine pneumococcal pneumonia. Exp Gerontol. 2012;47(7):507-518.
[DOI] -
33. Wynn TA, Chawla A, Pollard JW. Macrophage biology in development, homeostasis and disease. Nature. 2013;496(7446):445-455.
[DOI] -
34. Davies LC, Jenkins SJ, Allen JE, Taylor PR. Tissue-resident macrophages. Nat Immunol. 2013;14(10):986-995.
[DOI] -
35. Gibbings SL, Thomas SM, Atif SM, McCubbrey AL, Desch AN, Danhorn T, et al. Three Unique Interstitial Macrophages in the Murine Lung at Steady State. Am J Resp Cell Mol. 2017;57(1):66-76.
[DOI] -
36. Nagelkerke SQ, Bruggeman CW, den Haan JMM, Mul EPJ, van den Berg TK, van Bruggen R, et al. Red pulp macrophages in the human spleen are a distinct cell population with a unique expression of Fc-γ receptors. Blood Adv. 2018;2(8):941-953.
[DOI] -
37. Mass E, Nimmerjahn F, Kierdorf K, Schlitzer A. Tissue-specific macrophages: how they develop and choreograph tissue biology. Nat Rev Immunol. 2023;23:563-579.
[DOI] -
38. Cortez-Retamozo V, Etzrodt M, Newton A, Rauch PJ, Chudnovskiy A, Berger C, et al. Origins of tumor-associated macrophages and neutrophils. Proc Nat Acad Sci. 2012;109(7):2491-2496.
[DOI] -
39. Cortez-Retamozo V, Etzrodt M, Newton A, Ryan R, Pucci F, Sio SW, et al. Angiotensin II drives the production of tumor-promoting macrophages. Immunity. 2013;38(2):296-308.
[DOI] -
40. Swirski FK, Nahrendorf M, Etzrodt M, Wildgruber M, Cortez-Retamozo V, Panizzi P, et al. Identification of splenic reservoir monocytes and their deployment to inflammatory sites. Science. 2009;325(5940):612-616.
[DOI] -
41. Merad M, Manz MG, Karsunky H, Wagers A, Peters W, Charo I, et al. Langerhans cells renew in the skin throughout life under steady-state conditions. Nat Immunol. 2002;3(12):1135-1141.
[DOI] -
42. Hashimoto D, Chow A, Noizat C, Teo P, Beasley MB, Leboeuf M, et al. Tissue-Resident Macrophages Self-Maintain Locally throughout Adult Life with Minimal Contribution from Circulating Monocytes. Immunity. 2013;38(4):792-804.
[DOI] -
43. Hoeffel G, Wang Y, Greter M, See P, Teo P, Malleret B, et al. Adult Langerhans cells derive predominantly from embryonic fetal liver monocytes with a minor contribution of yolk sac–derived macrophages. J Exp Med. 2012;209(6):1167-1181.
[DOI] -
44. Ide S, Yahara Y, Kobayashi Y, Strausser SA, Ide K, Watwe A, et al. Yolk-sac-derived macrophages progressively expand in the mouse kidney with age. Elife. 2020;9:e51756-e51756.
[DOI] -
45. Dick S, Wong A, Hamidzada H, Nejat S, Nechanitzky R, Vohra S, et al. Three tissue resident macrophage subsets coexist across organs with conserved origins and life cycles. Sci Immunol. 2022;7(67):eabf7777-eabf7777.
[DOI] -
46. Laar L, Saelens W, De Prijck S, Martens L, Scott CL, Isterdael GV, et al. Yolk Sac Macrophages, Fetal Liver, and Adult Monocytes Can Colonize an Empty Niche and Develop into Functional Tissue-Resident Macrophages. Immunity. 2016;44(4):755-768.
[DOI] -
47. Weinberger T, Esfandyari D, Messerer D, Percin G, Schleifer C, Thaler R, et al. Ontogeny of arterial macrophages defines their functions in homeostasis and inflammation. Nat Commun. 2020;11(1):4549-4549.
[DOI] -
48. Wang X, Sathe AA, Smith GR, Ruf-Zamojski F, Nair V, Lavine KJ, et al. Heterogeneous origins and functions of mouse skeletal muscle-resident macrophages. Proc Nat Acad Sci. 2020;117(34):20729-20740.
[DOI] -
49. Murray PJ, Allen JE, Biswas SK, Fisher EA, Gilroy DW, Goerdt S, et al. Macrophage activation and polarization: nomenclature and experimental guidelines. Immunity. 2014;41(1):14-20.
[DOI] -
50. Unuvar Purcu D, Korkmaz A, Gunalp S, Helvaci DG, Erdal Y, Dogan Y, et al. Effect of stimulation time on the expression of human macrophage polarization markers. Plos One. 2022;17(3):e0265196-e0265196.
[DOI] -
51. Brecht K, Weigert A, Hu J, Popp R, Fisslthaler B, Korff T, et al. Macrophages programmed by apoptotic cells promote angiogenesis via prostaglandin E2. Faseb J. 2011;25(7):2408-2417.
[DOI] -
52. Graney P, Ben-Shaul S, Landau S, Bajpai A, Singh B, Eager J, et al. Macrophages of diverse phenotypes drive vascularization of engineered tissues. Sci Adv. 2020;6(18):eaay6391-eaay6391.
[DOI] -
53. Das A, Ganesh K, Khanna S, Sen CK, Roy S. Engulfment of Apoptotic Cells by Macrophages: A Role of MicroRNA-21 in the Resolution of Wound Inflammation. J Immunol. 2014;192(3):1120-1129.
[DOI] -
54. Arnold L, Henry A, Poron F, Baba-Amer Y, van Rooijen N, Plonquet A, et al. Inflammatory monocytes recruited after skeletal muscle injury switch into antiinflammatory macrophages to support myogenesis. J Exp Med. 2007;204(5):1057-1069.
[DOI] -
55. Svedberg FR, Brown SL, Krauss MZ, Campbell L, Sharpe C, Clausen M, et al. The lung environment controls alveolar macrophage metabolism and responsiveness in type 2 inflammation. Nat Immunol. 2019;20(5):571-580.
[DOI] -
56. Jackaman C, Yeoh TL, Acuil ML, Gardner JK, Nelson DJ. Murine mesothelioma induces locally-proliferating IL-10(+)TNF-α(+)CD206(-)CX3CR1(+) M3 macrophages that can be selectively depleted by chemotherapy or immunotherapy. Oncoimmunology. 2016;5(6):e1173299-e1173299.
[DOI] -
57. Boibessot C, Molina O, Lachance G, Tav C, Champagne A, Neveu B, et al. Subversion of infiltrating prostate macrophages to a mixed immunosuppressive tumor-associated macrophage phenotype. Clin Transl Med. 2022;12(1):e581-e581.
[DOI] -
58. Stout RD, Jiang C, Matta B, Tietzel I, Watkins SK, Suttles J. Macrophages sequentially change their functional phenotype in response to changes in microenvironmental influences. J Immunol. 2005;175(1):342-349.
[DOI] -
59. He H, Xu J, Warren CM, Duan D, Li X, Wu L, et al. Endothelial cells provide an instructive niche for the differentiation and functional polarization of M2-like macrophages. Blood. 2012;120(15):3152-3162.
[DOI] -
60. Leach HG, Chrobak I, Han R, Trojanowska M. Endothelial cells recruit macrophages and contribute to a fibrotic milieu in bleomycin lung injury. Am J Respir Cell Mol Biol. 2013;49(6):1093-1101.
[DOI] -
61. Mysore V, Tahir S, Furuhashi K, Arora J, Rosetti F, Cullere X, et al. Monocytes transition to macrophages within the inflamed vasculature via monocyte CCR2 and endothelial TNFR2. J Exp Med. 2022;219(5):e20210562-e20210562.
[DOI] -
62. Bruce AC, Kelly-Goss MR, Heuslein JL, Meisner JK, Price RJ, Peirce SM. Monocytes Are Recruited From Venules During Arteriogenesis in the Murine Spinotrapezius Ligation Model. Arterioscler Thromb Vasc Biol. 2014;34(9):2012-2022.
[DOI] -
63. Krishnasamy K, Limbourg A, Kapanadze T, Gamrekelashvili J, Beger C, Häger C, et al. Blood vessel control of macrophage maturation promotes arteriogenesis in ischemia. Nat Commun. 2017;8(1):952-952.
[DOI] -
64. Zhang J, Muri J, Fitzgerald G, Gorski T, Gianni-Barrera R, Masschelein E, et al. Endothelial Lactate Controls Muscle Regeneration from Ischemia by Inducing M2-like Macrophage Polarization. Cell Metab. 2020;31(6):1136-1153.
[DOI] -
65. Zhou B, Magana L, Hong Z, Huang LS, Chakraborty S, Tsukasaki Y, et al. The angiocrine Rspondin3 instructs interstitial macrophage transition via metabolic-epigenetic reprogramming and resolves inflammatory injury. Nat Immunol. 2020;21(11):1430-1443.
[DOI] -
66. Fantin A, Vieira JM, Gestri G, Denti L, Schwarz Q, Prykhozhij S, et al. Tissue macrophages act as cellular chaperones for vascular anastomosis downstream of VEGF-mediated endothelial tip cell induction. Blood. 2010;116(5):829-840.
[DOI] -
67. Gurevich DB, Severn CE, Twomey C, Greenhough A, Cash J, Toye AM, et al. Live imaging of wound angiogenesis reveals macrophage orchestrated vessel sprouting and regression. Embo Journal. 2018;37(13):e97786-e97786.
[DOI] -
68. Martin P, Gurevich DB. Macrophage regulation of angiogenesis in health and disease. Semin Cell Dev Biol. 2021;119:101-110.
[DOI] -
69. Willenborg S, Lucas T, van Loo G, Knipper JA, Krieg T, Haase I, et al. CCR2 recruits an inflammatory macrophage subpopulation critical for angiogenesis in tissue repair. Blood. 2012;120(3):613-625.
[DOI] -
70. Sainson RCA, Johnston DA, Chu HC, Holderfield MT, Nakatsu MN, Crampton SP, et al. TNF primes endothelial cells for angiogenic sprouting by inducing a tip cell phenotype. Blood. 2008;111(10):4997-5007.
[DOI] -
71. Spiller KL, Anfang RR, Spiller KJ, Ng J, Nakazawa KR, Daulton JW, et al. The role of macrophage phenotype in vascularization of tissue engineering scaffolds. Biomaterials. 2014;35(15):4477-4488.
[DOI] -
72. Puranik AS, Leaf IA, Jensen MA, Hedayat AF, Saad A, Kimet KW, et al. Kidney-resident macrophages promote a proangiogenic environment in the normal and chronically ischemic mouse kidney. Sci Rep. 2018;8(1):13948-13948.
[DOI] -
73. Dube CT, Ong YHB, Wemyss K, Krishnan S, Tan TJ, Janela B, et al. Age-Related Alterations in Macrophage Distribution and Function Are Associated With Delayed Cutaneous Wound Healing. Front Immunol. 2022;13:943159-943159.
[DOI] -
74. Chen Y, Hao X, Li M, Tian Z, Cheng M. UGRP1-modulated MARCO+ alveolar macrophages contribute to age-related lung fibrosis. Immun Ageing. 2023;20(1):14-14.
[DOI] -
75. Wong CK, Smith CA, Sakamoto K, Kaminski N, Koff JL, Goldstein DR. Aging impairs alveolar macrophage phagocytosis and increases influenza-induced mortality in mice. J Immunol. 2017;199(3):1060-1068.
[DOI] -
76. McQuattie-Pimentel AC, Ren Z, Joshi N, Watanabe S, Stoeger T, Chi M, et al. The lung microenvironment shapes a dysfunctional response of alveolar macrophages in aging. J Clin Invest. 2021;131(4):e140299-e140299.
[DOI] -
77. Stranks AJ, Hansen AL, Panse I, Mortensen M, Ferguson DJP, Puleston DJ, et al. Autophagy controls acquisition of aging features in macrophages. J Innate Immun. 2015;7(4):375-391.
[DOI] -
78. Lumeng CN, Liu J, Geletka L, Delaney C, Delproposto J, Desai A, et al. Aging is associated with an increase in T cells and inflammatory macrophages in visceral adipose tissue. J Immunol. 2011;187(12):6208-6216.
[DOI] -
79. Ahmadi M, Karlsen A, Mehling J, Soendenbroe C, Mackey AL, Hyldahl RD, et al. Aging is associated with an altered macrophage response during human skeletal muscle regeneration. Exp Gerontol. 2022;169:111974-111974.
[DOI] -
80. Tam C, Sparks L, Johannsen D, Covington JD, Church TS, Ravussin E. Low macrophage accumulation in skeletal muscle of obese type 2 diabetics and elderly subjects. Obesity. 2012;20(7):1530-1533.
[DOI] -
81. Jensen SM, Bechshøft CJL, Heisterberg MF, Schjerling P, Andersen JL, Kjaer M, et al. Macrophage subpopulations and the acute inflammatory response of elderly human skeletal muscle to physiological resistance exercise. Front Physiol. 2020;11:811-811.
[DOI] -
82. Cui CY, Driscoll RK, Piao Y, Chia CW, Gorospe M, Ferrucci L. Skewed macrophage polarization in aging skeletal muscle. Aging Cell. 2019;18(6):e13032-e13032.
[DOI] -
83. Chittimalli K, Jahan J, Sakamuri A, Weyrick H, Winkle W, Adkins S, et al. Reversal of aging-associated increase in myelopoiesis and expression of alarmins by angiotensin-(1-7). Sci Rep. 2023;13(1):2543-2543.
[DOI] -
84. Duong L, Pixley FJ, Nelson DJ, Jackaman C. Aging Leads to Increased Monocytes and Macrophages With Altered CSF-1 Receptor Expression and Earlier Tumor-Associated Macrophage Expansion in Murine Mesothelioma. Front Aging. 2022;3:848925-848925.
[DOI] -
85. Jackaman C, Radley-Crabb HG, Soffe Z, Shavlakadze T, Grounds MD, Nelson DJ. Targeting macrophages rescues age-related immune deficiencies in C57BL/6J geriatric mice. Aging Cell. 2013;12(3):345-357.
[DOI] -
86. Kim OH, Kim H, Kang J, Yang D, Kang YH, Lee DH, et al. Impaired phagocytosis of apoptotic cells causes accumulation of bone marrow-derived macrophages in aged mice. BMB Rep. 2017;50(1):43-48.
[DOI] -
87. Bloomer SA, Moyer ED, Brown KE, Kregel KC. Aging results in accumulation of M1 and M2 hepatic macrophages and a differential response to gadolinium chloride. Histochem Cell Biol. 2020;153(1):37-48.
[DOI] -
88. Hilmer SN, Cogger VC, Le Couteur DG. Basal activity of Kupffer cells increases with old age. J Gerontol A. 2007;62(9):973-978.
[DOI] -
89. Golden-Mason L, O'Farrelly C. Having it all? Stem cells, haematopoiesis and lymphopoiesis in adult human liver. Immunol Cell Biol. 2002;80(1):45-51.
[DOI] -
90. Costantini A, Viola N, Berretta A, Galeazzi R, Matacchione G, Sabbatinelli J, et al. Age-related M1/M2 phenotype changes in circulating monocytes from healthy/unhealthy individuals. Aging. 2018;10(6):1268-1280.
[DOI] -
91. Cao Y, Fan Y, Li F, Hao Y, Kong Y, Chen C, et al. Phenotypic and functional alterations of monocyte subsets with aging. Immun Ageing. 2022;19(1):63-63.
[DOI] -
92. Seidler S, Zimmermann HW, Bartneck M, Trautwein C, Tacke F. Age-dependent alterations of monocyte subsets and monocyte-related chemokine pathways in healthy adults. BMC Immunol. 2010;11(1):30-30.
[DOI] -
93. Ho TT, Warr MR, Adelman ER, Lansinger OM, Flach J, Verovskaya EV, et al. Autophagy maintains the metabolism and function of young and old stem cells. Nature. 2017;543(7644):205-210.
[DOI] -
94. Dykstra B, Olthof S, Schreuder J, Ritsema M, de Haan G. Clonal analysis reveals multiple functional defects of aged murine hematopoietic stem cells. J Exp Med. 2011;208(13):2691-2703.
[DOI] -
95. Cho RH, Sieburg HB, Muller-Sieburg CE. A new mechanism for the aging of hematopoietic stem cells: aging changes the clonal composition of the stem cell compartment but not individual stem cells. Blood. 2008;111(12):5553-5561.
[DOI] -
96. Pang WW, Price EA, Sahoo D, Beerman I, Maloney WJ, Rossi DJ, et al. Human bone marrow hematopoietic stem cells are increased in frequency and myeloid-biased with age. Proc Nat Acad Sci. 2011;108(50):20012-20017.
[DOI] -
97. Linehan E, Dombrowski Y, Snoddy R, Fallon PG, Kissenpfennig A, Fitzgerald DC. Aging impairs peritoneal but not bone marrow-derived macrophage phagocytosis. Aging Cell. 2014;13(4):699-708.
[DOI] -
98. Wang Y, Wehling-Henricks M, Samengo G, Tidball JG. Increases of M2a macrophages and fibrosis in aging muscle are influenced by bone marrow aging and negatively regulated by muscle-derived nitric oxide. Aging Cell. 2015;14(4):678-688.
[DOI] -
99. Frisch BJ, Hoffman CM, Latchney SE, LaMere MW, Myers J, Ashton J, et al. Aged marrow macrophages expand platelet-biased hematopoietic stem cells via interleukin-1B. JCI Insight. 2019;5(10):e124213-e124213.
[DOI] -
100. Pinke KH, Calzavara B, Faria PF, do Nascimento MP, Venturini J, Lara VS. Proinflammatory profile of in vitro monocytes in the ageing is affected by lymphocytes presence. Immun Ageing. 2013;10(1):22-22.
[DOI] -
101. Becker L, Nguyen L, Gill J, Kulkarni S, Pasricha PJ, Habtezion A. Age-dependent shift in macrophage polarisation causes inflammation-mediated degeneration of enteric nervous system. Gut. 2018;67(5):827-836.
[DOI] -
102. Brandenberger C, Kling KM, Vital M, Mühlfeld C. The Role of Pulmonary and Systemic Immunosenescence in Acute Lung Injury. Aging Dis. 2018;9(4):553-565.
[DOI] -
103. Renshaw M, Rockwell J, Engleman C, Gewirtz A, Katz J, Sambhara S. Cutting Edge: Impaired Toll-Like Receptor Expression and Function in Aging. J Immunol. 2002;169(9):4697-4701.
[DOI] -
104. Boehmer ED, Goral J, Faunce DE, Kovacs EJ. Age-dependent decrease in Toll-like receptor 4-mediated proinflammatory cytokine production and mitogen-activated protein kinase expression. J Leukocyte Biol. 2004;75(2):342-349.
[DOI] -
105. Duin D, Mohanty S, Thomas V, Ginter S, Montgomery RR, Fikrig E, et al. Age-Associated Defect in Human TLR-1/2 Function. J Immunol. 2007;178(2):970-975.
[DOI] -
106. Nyugen J, Agrawal S, Gollapudi S, Gupta S. Impaired Functions of Peripheral Blood Monocyte Subpopulations in Aged Humans. J Clin Immunol. 2010;30(6):806-813.
[DOI] -
107. Chelvarajan RL, Collins SM, Van Willigen JM, Bondada S. The unresponsiveness of aged mice to polysaccharide antigens is a result of a defect in macrophage function. J Leukocyte Biol. 2005;77(4):503-512.
[DOI] -
108. Wu D, Mura C, Beharka AA, Han SN, Paulson KE, Hwang D, et al. Age-associated increase in PGE2 synthesis and COX activity in murine macrophages is reversed by vitamin E. Am J Physiol-cell Ph. 1998;275(3):C661-C668.
[DOI] -
109. Herrero C, Marqués L, Lloberas J, Celada A. IFN-γ–dependent transcription of MHC class II IA is impaired in macrophages from aged mice. J Clin Invest. 2001;107(4):485-493.
[DOI] -
110. Jämsen E, Pajarinen J, Lin TH, Lo CW, Nabeshima A, Lu L, et al. Effect of Aging on the Macrophage Response to Titanium Particles. J Orthop Res. 2020;38(2):405-416.
[DOI] -
111. Tomay F, Wells K, Duong L, Tsu JW, Dye DE, Radley-Crabb HG, et al. Aged neutrophils accumulate in lymphoid tissues from healthy elderly mice and infiltrate T- and B-cell zones. Immunol Cell Biol. 2018;96(8):831-840.
[DOI] -
112. Martin G, Sewell RB, Yeomans ND, Morgan DJ, Smallwood RA. Hepatic Kupffer cell function: the efficiency of uptake and intracellular degradation of 14C-labelled mitochondria is reduced in aged rats. Mech Ageing Dev. 1994;73(3):157-168.
[DOI] -
113. Durham SK, Brouwer A, Barelds RJ, Horan MA, Knook DL. Comparative endotoxin-induced hepatic injury in young and aged rats. J Pathol. 1990;162(4):341-349.
[DOI] -
114. A-Gonzalez N, Quintana JA, García-Silva S, Mazariegos M, González de la Aleja A, Nicolas-Avila JA, et al. Phagocytosis imprints heterogeneity in tissue-resident macrophages. J Exp Med. 2017;214(5):1281-1296.
[DOI] -
115. Zhang S, Weinberg S, DeBerge M, Gainullina A, Schipma M, Kinchen JM, et al. Efferocytosis Fuels Requirements of Fatty Acid Oxidation and the Electron Transport Chain to Polarize Macrophages for Tissue Repair. Cell Metab. 2019;29(2):443-456.
[DOI] -
116. Yousefzadeh M, Flores R, Zhu Y, Schmiechen ZC, Brooks RW, Trussoni CE, et al. An aged immune system drives senescence and ageing of solid organs. Nature. 2021;594(7861):100-105.
[DOI] -
117. Yousefzadeh MJ, Zhao J, Bukata C, Wade EA, McGowan SJ, Angelini LA, et al. Tissue specificity of senescent cell accumulation during physiologic and accelerated aging of mice. Aging Cell. 2020;19(3):e13094-e13094.
[DOI] -
118. Ovadya Y, Landsberger T, Leins H, Vadai E, Gal H, Biran A, et al. Impaired immune surveillance accelerates accumulation of senescent cells and aging. Nat Commun. 2018;9(1):5435-5435.
[DOI] -
119. Liu Z, Gu Y, Chakarov S, Bleriot C, Kwok I, Chen X, et al. Fate Mapping via Ms4a3-Expression History Traces Monocyte-Derived Cells. Cell. 2019;178(6):1509-1525.
[DOI] -
120. Mariano LL, Rousseau M, Varet H, Legendre R, Gentek R, Coronilla JS, et al. Functionally distinct resident macrophage subsets differentially shape responses to infection in the bladder. Sci Adv. 2020;6(48):eabc5739-eabc5739.
[DOI] -
121. Misharin AV, Morales-Nebreda L, Reyfman PA, Cuda CM, Walter JM, McQuattie-Pimentel AC, et al. Monocyte-derived alveolar macrophages drive lung fibrosis and persist in the lung over the life span. J Exp Med. 2017;214(8):2387-2404.
[DOI] -
122. Gather L, Nath N, Falckenhayn C, Oterino-Sogo S, Bosch T, Wenck H, et al. Macrophages Are Polarized toward an Inflammatory Phenotype by their Aged Microenvironment in the Human Skin. J Invest Dermatol. 2022;142(12):3136-3145.
[DOI] -
123. Zhang Z, Schlamp F, Huang L, Clark H, Brayboy L. Inflammaging is associated with shifted macrophage ontogeny and polarization in the aging mouse ovary. Reproduction. 2020;159(3):325-337.
[DOI] -
124. Molawi K, Wolf Y, Kandalla PK, Favret J, Hagemeyer N, Frenzel K, et al. Progressive replacement of embryo-derived cardiac macrophages with age. J Exp Med. 2014;211(11):2151-2158.
[DOI] -
125. Duong L, Radley-Crabb HG, Gardner JK, Tomay F, Dye DE, Grounds MD, et al. Macrophage Depletion in Elderly Mice Improves Response to Tumor Immunotherapy, Increases Anti-tumor T Cell Activity and Reduces Treatment-Induced Cachexia. Front Genet. 2018;9:526-526.
[DOI] -
126. Cao LL, Che XF, Qiu XS, Li Z, Yang BW, Wang S, et al. M2 macrophage infiltration into tumor islets leads to poor prognosis in non-small-cell lung cancer. Cancer Manag Res. 2019;11:6125-6138.
[DOI] -
127. Zhang B, Yao G, Zhang Y, Gao J, Yang B, Rao Z, et al. M2-Polarized tumor-associated macrophages are associated with poor prognoses resulting from accelerated lymphangiogenesis in lung adenocarcinoma. Clinics. 2011;66(11):1879-1886.
[DOI] -
128. Sanchez-Moral L, Paul T, Martori C, Font-Díaz J, Sanjurjoa L, Aran G, et al. Macrophage CD5L is a target for cancer immunotherapy. eBioMedicine 2023;91:104555-104555.
[DOI] -
129. Franklin R, Liao W, Sarkar A, Kim MV, Bivona MR, Liu K, et al. The cellular and molecular origin of tumor-associated macrophages. Science. 2014;344(6186):921-925.
[DOI] -
130. Proctor DT, Huang J, Lama S, Albakr A, Van Marle, Sutherland GR. Neuro-Oncology Advances 2019;1(1):vdz018-vdz018.
[DOI] -
131. Bianchi-Frias D, Damodarasamy M, Hernandez SA, Gil da Costa RM, Vakar-Lopez F, Coleman IM, et al. The aged microenvironment influences the tumorigenic potential of malignant prostate epithelial cells. Mol Cancer Res. 2019;17(1):321-331.
[DOI] -
132. Chen JJ, Lin YC, Yao PL, Yuan A, Chen HY, Shun CT, et al. Tumor-associated macrophages: the double-edged sword in cancer progression. J Clin Oncol. 2005;23(5):953-964.
[DOI] -
133. Zhu XD, Zhang JB, Zhuang PY, Zhu HG, Zhang W, Xiong YQ, et al. High expression of macrophage colony-stimulating factor in peritumoral liver tissue is associated with poor survival after curative resection of hepatocellular carcinoma. J Clin Oncol. 2008;26(16):2707-2716.
[DOI] -
134. Zhao X, Qu J, Sun Y, Wang J, Liu X, Wang F, et al. Prognostic significance of tumor-associated macrophages in breast cancer: a meta-analysis of the literature. Oncotarget. 2017;8(18):30576-30586.
[DOI] -
135. Casanova-Acebes M, Dalla E, Leader AM, LeBerichel J, Nikolic J, Morales BM, et al. Tissue-resident macrophages provide a pro-tumorigenic niche to early NSCLC cells. Nature. 2021;595(7868):578-584.
[DOI] -
136. Liu L, Ye Y, Zhu X. MMP-9 secreted by tumor associated macrophages promoted gastric cancer metastasis through a PI3K/AKT/Snail pathway. Biomed Pharmacother. 2019;117:109096-109096.
[DOI] -
137. Arlauckas SP, Garris CS, Kohler RH, Kitaoka M, Cuccarese MF, Yang KS, et al. In vivo imaging reveals a tumor-associated macrophage-mediated resistance pathway in anti-PD-1 therapy. Sci Transl Med. 2017;9(389):eaal3604-eaal3604.
[DOI] -
138. Shree T, Olson OC, Elie BT, Kester JC, Garfall AL, Simpson K, et al. Macrophages and cathepsin proteases blunt chemotherapeutic response in breast cancer. Gene Dev. 2011;25(23):2465-2479.
[DOI] -
139. Quaranta V, Rainer C, Nielsen SR, Raymant ML, Ahmed MS, Engle DD, et al. Macrophage-derived granulin drives resistance to immune checkpoint inhibition in metastatic pancreatic cancer. Cancer Res. 2018;78(15):4253-4269.
[DOI] -
140. Modak M, Mattes AK, Reiss D, Skronska-Wasek W, Langlois R, Sabarth N, et al. CD206+ tumor-associated macrophages cross-present tumor antigen and drive antitumor immunity. JCI Insight. 2022;7(11):e155022-e155022.
[DOI] -
141. Munn DH, Cheung NK. Phagocytosis of tumor cells by human monocytes cultured in recombinant macrophage colony-stimulating factor. J Exp Med. 1990;172(1):231-237.
[DOI] -
142. Johnson WJ, Bolognesi DP, Adams DO. Antibody-dependent cytolysis (ADCC) of tumor cells by activated murine macrophages is a two-step process: Quantification of target binding and subsequent target lysis. Cell Immunol. 1984;83(1):170-180.
[DOI] -
143. Tsao LC, Crosby EJ, Trotter TN, Agarwal P, Hwang BJ, Acharya C, et al. CD47 blockade augmentation of trastuzumab antitumor efficacy dependent on antibody-dependent cellular phagocytosis. JCI Insight. 2019;4(24):e131882-e131882.
[DOI] -
144. Mantovani A, Sica A. Macrophages, innate immunity and cancer: balance, tolerance, and diversity. Curr Opin Immunol. 2010;22(2):231-237.
[DOI] -
145. Hirano R, Okamoto K, Shinke M, Sato M, Watanabe S, Watanabe H, et al. Tissue-resident macrophages are major tumor-associated macrophage resources, contributing to early TNBC development, recurrence, and metastases. Commun Biol. 2023;6(1):144-144.
[DOI] -
146. Dirkx AE, Oude Egbrink MG, Wagstaff J, Griffioen AW. Monocyte/macrophage infiltration in tumors: modulators of angiogenesis. J Leukocyte Biol. 2006;80(6):1183-1196.
[DOI] -
147. Dwyer AR, Greenland EL, Pixley FJ. Promotion of tumor invasion by tumor-associated macrophages: the role of CSF-1-activated phosphatidylinositol 3 kinase and Src family kinase motility signaling. Cancers. 2017;9(6):68-68.
[DOI] -
148. Gyori D, Lim EL, Grant FM, Spensberger D, Roychoudhuri R, Shuttleworth SJ, et al. Compensation between CSF1R+ macrophages and Foxp3+ treg cells drives resistance to tumor immunotherapy. JCI Insight. 2018;3(11):e120631-e120631.
[DOI] -
149. Sanford DE, Belt BA, Panni RZ, Mayer A, Deshpande AD, Carpenter D, et al. Inflammatory monocyte mobilization decreases patient survival in pancreatic cancer: a role for targeting the CCL2/CCR2 axis. Clin Cancer Res. 2013;19(13):3404-3415.
[DOI] -
150. Walens A, DiMarco AV, Lupo R, Kroger BR, Damrauer JS, Alvarez JV. CCL5 promotes breast cancer recurrence through macrophage recruitment in residual tumors. Elife. 2019;8:e43653-e43653.
[DOI] -
151. Okikawa S, Morine Y, Saito Y, Yamada S, Tokuda K, Teraoku H, et al. Inhibition of the VEGF signaling pathway attenuates tumor‑associated macrophage activity in liver cancer. Oncol Rep. 2022;47(4):71-71.
[DOI] -
152. Linde N, Lederle W, Depner S, van Rooijen N, Gutschalk CM, Mueller MM. Vascular endothelial growth factor-induced skin carcinogenesis depends on recruitment and alternative activation of macrophages. J Pathol. 2012;227(1):17-28.
[DOI] -
153. Standiford TJ, Kuick R, Bhan U, Chen J, Newstead M, Keshamouni VG. TGF-β-induced IRAK-M expression in tumor-associated macrophages regulates lung tumor growth. Oncogene. 2011;30(21):2475-2484.
[DOI] -
154. Shen H, Liu J, Chen S, Ma X, Ying Y, Li J, et al. Prognostic value of tumor-associated macrophages in clear cell renal cell carcinoma: a systematic review and meta-analysis. Front Oncol. 2021;11:657318-657318.
[DOI] -
155. Wang Q, He Z, Huang M, Liu T, Wang Y, Xu H, et al. Vascular niche IL-6 induces alternative macrophage activation in glioblastoma through HIF-2α. Nat Commun. 2018;9(1):559-559.
[DOI] -
156. Yin S, Huang J, Li Z, Zhang J, Luo J, Lu C, et al. The prognostic and clinicopathological significance of tumor-associated macrophages in patients with gastric cancer: a meta-analysis. Plos One. 2017;12(1):e0170042-e0170042.
[DOI] -
157. Gordon SR, Maute RL, Dulken BW, Hutter G, George BM, McCracken MN, et al. PD-1 expression by tumour-associated macrophages inhibits phagocytosis and tumour immunity. Nature. 2017;545(7655):495-499.
[DOI] -
158. Helm O, Held-Feindt J, Grage-Griebenow E, Reiling N, Ungefroren H, Vogel I, et al. Tumor-associated macrophages exhibit pro- and anti-inflammatory properties by which they impact on pancreatic tumorigenesis. Int J Cancer. 2014;135(4):843-861.
[DOI] -
159. Chen D, Varanasi SK, Hara T, Traina K, Sun M, McDonald B, et al. CTLA-4 blockade induces a microglia-Th1 cell partnership that stimulates microglia phagocytosis and anti-tumor function in glioblastoma. Immunity. 2023;56(9):2086-2104.
[DOI] -
160. Romano E, Kusio-Kobialka M, Foukas PG, Baumgaertner P, Meyer C, Ballabeni P, et al. Ipilimumab-dependent cell-mediated cytotoxicity of regulatory T cells ex vivo by nonclassical monocytes in melanoma patients. Proc Nat Acad Sci. 2015;112(19):6140-6145.
[DOI] -
161. Rashidian M, LaFleur MW, Verschoor VL, Dongre A, Zhang Y, Nguyen TH, et al. Immuno-PET identifies the myeloid compartment as a key contributor to the outcome of the antitumor response under PD-1 blockade. Proc Nat Acad Sci. 2019;116(34):16971-16980.
[DOI] -
162. Peranzoni E, Lemoine J, Vimeux L, Feuillet V, Barrin S, Kantari-Mimoun C, et al. Macrophages impede CD8 T cells from reaching tumor cells and limit the efficacy of anti–PD-1 treatment. Proc Nat Acad Sci. 2018;115(17):E4041-E4050.
[DOI] -
163. Pombo Antunes AR, Scheyltjens I, Lodi F, Messiaen J, Antoranz A, Duerinck J, et al. Single-cell profiling of myeloid cells in glioblastoma across species and disease stage reveals macrophage competition and specialization. Nat Neurosci. 2021;24(4):595-610.
[DOI] -
164. Loyher PL, Hamon P, Laviron M, Meghraoui-Kheddar A, Goncalves E, Deng Z, et al. Macrophages of distinct origins contribute to tumor development in the lung. J Exp Med. 2018;215(10):2536-2553.
[DOI] -
165. Simon MP, Tournaire R, Pouyssegur J. The angiopoietin-2 gene of endothelial cells is up-regulated in hypoxia by a HIF binding site located in its first intron and by the central factors GATA-2 and Ets-1. J Cell Physiol. 2008;217(3):809-818.
[DOI] -
166. Pichiule P, Chavez JC, LaManna JC. Hypoxic regulation of angiopoietin-2 expression in endothelial cells. J Biol Chem. 2004;279(13):12171-12180.
[DOI] -
167. Murdoch C, Giannoudis A, Lewis CE. Mechanisms regulating the recruitment of macrophages into hypoxic areas of tumors and other ischemic tissues. Blood. 2004;104(8):2224-2234.
[DOI] -
168. Gopinathan G, Milagre C, Pearce OM, Reynolds LE, Hodivala-Dilke K, Leinster DA, et al. Interleukin-6 stimulates defective angiogenesis. Cancer Res. 2015;75(15):3098-3107.
[DOI] -
169. Bonapace L, Coissieux MM, Wyckoff J, Mertz KD, Varga Z, Junt T, et al. Cessation of CCL2 inhibition accelerates breast cancer metastasis by promoting angiogenesis. Nature. 2014;515(7525):130-133.
[DOI] -
170. Giraudo E, Inoue M, Hanahan D. An amino-bisphosphonate targets MMP-9–expressing macrophages and angiogenesis to impair cervical carcinogenesis. J Clin Invest. 2004;114(5):623-633.
[DOI] -
171. Henze AT, Mazzone M. The impact of hypoxia on tumor-associated macrophages. J Clin Invest. 2016;126(10):3672-3679.
[DOI] -
172. Coffelt SB, Tal AO, Scholz A, de Palma M, Patel S, Urbich C, et al. Angiopoietin-2 regulates gene expression in TIE2-expressing monocytes and augments their inherent proangiogenic functions. Cancer Res. 2010;70(13):5270-5280.
[DOI] -
173. Mazzieri R, Pucci F, Moi D, Zonari E, Ranghetti A, Berti A, et al. Targeting the ANG2/TIE2 axis inhibits tumor growth and metastasis by impairing angiogenesis and disabling rebounds of proangiogenic myeloid cells. Cancer Cell. 2011;19(4):512-526.
[DOI] -
174. Bianchi-Frias D, Vakar-Lopez F, Coleman IM, Plymate SR, Reed MJ, Nelson PS. The effects of aging on the molecular and cellular composition of the prostate microenvironment. Plos One. 2010;5(9):e12501-e12501.
[DOI] -
175. Li YN, Zhao YH, Gao Y, Li Y, Liu M, Xu NZ, et al. Age-related macrophage alterations are associated with carcinogenesis of colorectal cancer. Carcinogenesis. 2022;43(11):1039-1049.
[DOI] -
176. Teixeira LR, Almeida LY, Silva RN, Mesquita ATM, Colturato CBN, Silveira HA, et al. Young and elderly oral squamous cell carcinoma patients present similar angiogenic profile and predominance of M2 macrophages: Comparative immunohistochemical study. Head Neck. 2019;41(12):4111-4120.
[DOI] -
177. Wallace PK, Eisenstein TK, Meissler JJ, Morahan PS. Decreases in macrophage mediated antitumor activity with aging. Mech Ageing Dev. 1995;77(3):169-184.
[DOI] -
178. Plowden J, Renshaw-Hoelscher M, Gangappa S, Engleman C, Katz JM, Sambhara S. Impaired antigen-induced CD8+ T cell clonal expansion in aging is due to defects in antigen presenting cell function. Cell Immunol. 2004;229(2):86-92.
[DOI] -
179. Ma L, Li K, Wei W, Zhou J, Li Z, Zhang T, et al. Exercise protects aged mice against coronary endothelial senescence via FUNDC1-dependent mitophagy. Redox Biol. 2023;62:102693-102693.
[DOI] -
180. Chen B, Sun YJ, Zhang JT, Zhu QW, Yang YL, Niu X, et al. Human embryonic stem cell-derived exosomes promote pressure ulcer healing in aged mice by rejuvenating senescent endothelial cells. Stem Cell Res Ther. 2019;10(1):142-142.
[DOI] -
181. Li Y, Kracun D, Dustin CM, EI Massry M, Yuan S, Goossen CJ, et al. Forestalling age-impaired angiogenesis and blood flow by targeting NOX: Interplay of NOX1, IL-6, and SASP in propagating cell senescence. Proc Nat Acad Sci. 2021;118(42):e2015666118-e2015666118.
[DOI] -
182. Moriya J, Minamino T. Angiogenesis, cancer, and vascular aging. Front Cardiovasc Med. 2017;4:65-65.
[DOI] -
183. Bhayadia R, Schmidt BMW, Melk A, Hömme M. Senescence-induced oxidative stress causes endothelial dysfunction. J Gerontol A. 2015;71(2):161-169.
[DOI] -
184. Rossman MJ, Kaplon RE, Hill SD, McNamara MN, Santos-Parker JR, Pierce GL, et al. Endothelial cell senescence with aging in healthy humans: prevention by habitual exercise and relation to vascular endothelial function. Am J Physiol-Heart C. 2017;313(5):H890-H895.
[DOI] -
185. Rajapakse AG, Yepuri G, Carvas JM, Stein S, Matter CM, Scerri I, et al. Hyperactive S6K1 mediates oxidative stress and endothelial dysfunction in aging: inhibition by resveratrol. Plos One. 2011;6(4):e19237-e19237.
[DOI] -
186. Krouwer VJD, Hekking LHP, Langelaar-Makkinje M, Regan-Klapisz E, Post JA. Endothelial cell senescence is associated with disrupted cell-cell junctions and increased monolayer permeability. Vascular Cell. 2012;4(1):12-12.
[DOI] -
187. Lin JR, Shen WL, Yan C, Gao PJ. Downregulation of dynamin-related protein 1 contributes to impaired autophagic flux and angiogenic function in senescent endothelial cells. Arterioscl Throm Vasc Biol. 2015;35(6):1413-1422.
[DOI] -
188. Coppé JP, Desprez PY, Krtolica A, Campisi J. The Senescence-associated secretory phenotype: the dark side of tumor suppression. Annu Rev Pathol-Mech. 2010;5(1):99-118.
[DOI] -
189. Coleman PR, Chang G, Hutas G, Grimshaw M, Vadas MA, Gamble JR. Age-associated stresses induce an anti-inflammatory senescent phenotype in endothelial cells. Aging. 2013;5(12):913-924.
[DOI] -
190. Coleman PR, Hahn CN, Grimshaw M, Lu Y, Li X, Brautigan PJ, et al. Stress-induced premature senescence mediated by a novel gene, SENEX, results in an anti-inflammatory phenotype in endothelial cells. Blood. 2010;116(19):4016-4024.
[DOI] -
191. Powter EE, Coleman PR, Tran MH, Lay AJ, Bertolino P, Parton RG, et al. Caveolae control the anti-inflammatory phenotype of senescent endothelial cells. Aging Cell. 2015;14(1):102-111.
[DOI] -
192. Sharma C, Wang HX, Li Q, Knoblich K, Reisenbichler ES, Richardson AL, et al. Protein acyltransferase DHHC3 regulates breast tumor growth, oxidative stress, and senescence. Cancer Res. 2017;77(24):6880-6890.
[DOI] -
193. Haston S, Gonzalez-Gualda E, Morsli S, Ge J, Reen V, Calderwood A, et al. Clearance of senescent macrophages ameliorates tumorigenesis in KRAS-driven lung cancer. Cancer Cell. 2023;41(7):1242-1260.
[DOI] -
194. Ly L, Baghat A, Versluis M, Jordanova ES, Luyten GPM, van Rooijen N, et al. In aged mice, outgrowth of intraocular melanoma depends on proangiogenic M2-type macrophages. J Immunol. 2010;185(6):3481-3488.
[DOI]
Copyright
© The Author(s) 2024. This is an Open Access article licensed under a Creative Commons Attribution 4.0 International License (https://creativecommons.org/licenses/by/4.0/), which permits unrestricted use, sharing, adaptation, distribution and reproduction in any medium or format, for any purpose, even commercially, as long as you give appropriate credit to the original author(s) and the source, provide a link to the Creative Commons license, and indicate if changes were made.
Share And Cite