Abstract
Aims: The combination of CDK4/6 inhibitors and endocrine therapy (ET) is a standard first-line therapy for hormone receptor positive (HR+)/HER2- metastatic breast cancer (mBC). Preliminary data suggest that CDK4/6 inhibitors can alter the host immune function and stimulate tumor cell-directed immunity. However, clinical data are scarce, and no data exist about the impact of age and frailty on this phenomenon.
Materials and Methods: This biomarker substudy of the RibOB trial evaluated the impact of ribociclib and letrozole on circulating immune cell subsets and protein markers in older (≥ 70 years) patients with HR+/HER2-mBC. Peripheral blood mononuclear cell subtyping and analysis of plasma immune response and checkpoint markers were performed using flow cytometry at baseline and after three months of ribociclib + ET. Frailty status was assessed at baseline using G8 score.
Results: 20 patients (median age: 76 years, range: 70 - 87 years), 8 considered fit (G8 > 14), and 12 frail (G8 ≤ 14), were included. After three months of treatment, the immune subset composition showed significant increases in naïve B-, T-regulatory (Tregs), and CD4+ T-cells, while memory B-cells and Tregs were significantly decreased. In addition, consistent upregulation was seen in costimulatory receptors CD27 and CD28. Plasma immune checkpoint markers B7.2 (CD86) and PD-1 were significantly decreased. The immune subset profiles of fit versus frail persons showed no statistically significant difference.
Conclusions: The study shows that the combination of ribociclib and ET modulates the immune system in older patients, potentially reversing the age-related immunosenescence process by increasing naïve T-cell and B-cell populations and decreasing memory populations.
Clinical trial registration number: NCT03956654.
Keywords
1. Introduction
Breast cancer (BC) is considered an age-related disease. Due to the consistent increase in the proportion of older people in the last decades, aging biology and its interaction with cancer biology have emerged as novel areas of scientific interest[1,2].
Aging can be appraised in terms of biological changes in certain tissues, most particularly the blood compartment and immune system. Immunosenescence is the term used to describe the age-related decline in immune function that makes older people more vulnerable to infectious disease; it is thus associated with increased morbidity and mortality[3]. Although aging and cancer are closely intertwined and share many underlying mechanisms[3], no in-depth research has been performed on the aging-related immune modifications in the setting of (older) cancer patients. Declining immunological capacity and reduced immune surveillance are two common hypotheses to explain why cancer rates rise with age[2-6]. In addition, the aging process also seems to affect the biology of breast and other tumors. While triple-negative BC (TNBC) and human epidermal growth factor 2 (HER2)-positive BC appear to be more common in younger BC patients, hormone receptor-positive (HR+)/HER2-BC, also called luminal-like BC, is the most frequent BC subtype in older women[7-11].
When patients with luminal-like BC develop distant metastases, the disease becomes incurable. Endocrine therapy (ET) is the cornerstone of treating metastatic HR+/HER2- BC, but recent publications have shown that ET in combination with CDK4/6 inhibitors leads to major improvement of disease control and prolonged survival while generally maintaining quality of life[12-17]. Progression-free survival (PFS) was significantly extended when both an endocrine inhibitor and CDK4/6 inhibitor were administered concomitantly[12,13,18-20]. For instance, in the MONALEESA-2 study, which included only post-menopausal women with HR+/HER2- metastatic BC (mBC), first-line ribociclib together with letrozole resulted in a median PFS of 25.3 months, as opposed to 16.0 months for the control group receiving letrozole plus placebo, with a hazard ratio of 0.57 (95% CI: 0.46 - 0.70)[13]. This study convincingly demonstrated that CDK4/6 inhibitors confer therapeutic benefit to older persons as well. It should be noted, however, that pivotal trials in oncology do not include many frail older persons, even though these represent a major group among the older population[18,19].
CDK4/6 inhibitors induce cell cycle arrest in (breast) tumors through dephosphorylation of retinoblastoma (Rb), preventing cells to progress from G1 to S phase. CDK4/6 inhibitors also exert this cell cycle inhibitory effect in dividing non-cancer cells, such as white blood/immune cells, and can thus potentially affect anti-tumor immunity. Moreover, CDK4/6 inhibitors also show immunomodulatory effects, causing higher T-cell activation and depletion of T-regulatory cells after administration[16,21]. However, the effect of CDK4/6 inhibitors on the host immune system of the older patient, which was previously already shown to be remodeled due to immunosenescence, has not been studied yet[22].
The RibOB trial (NCT03956654) is a phase IV observational study evaluating the efficacy and tolerability of CDK4/6 inhibitor ribociclib in combination with letrozole, an aromatase inhibitor, in older (≥ 70 years) patients with HR+/HER2- mBC. In a preplanned biomarker substudy, we aimed to investigate the impact of this treatment on the immune system, by assessing treatment-induced alterations of peripheral blood mononuclear cell (PBMC) subsets and plasma immune response and immune checkpoint markers.
2. Materials and Methods
2.1 Study design and patient inclusion
The RibOB study (NCT03956654) is an observational prospective, open-label, single-arm, phase IV trial that evaluates the clinical efficacy, overall safety, and tolerability of ribociclib in combination with letrozole in older women (≥ 70 years) with HR+/HER2- mBC and no prior hormonal treatment for metastatic disease as per the indication approved by the European Medicines Authority (EMA) and as made available by Belgian national authorities in the national health care system. HER2-negative BC was defined by an immunohistochemistry (IHC) score of 0 or 1+ or a negative in situ hybridization with a score of 2+ or 3+ by IHC. IHC and in situ hybridization tests were performed by local laboratory testing according to the ASCO-CAP guidelines[23]. HR+ status was defined as histologically and/or cytologically confirmed diagnosis of estrogen receptor (ER) positive and/or progesterone receptor (PgR) positive BC (defined as ER and/or PgR ≥ 1% or Allred score > 2) by the local clinical laboratory. Patients had to have a baseline 12-lead ECG with QTcF at screening < 450 msec, adequate bone marrow and organ function, and had to be able to swallow ribociclib and letrozole tablets. Patients were excluded if they had previously received any CDK4/6 inhibitor, or systemic antihormonal therapy or chemotherapy for mBC. Patients with central nervous system (CNS) metastases were permitted if the CNS lesions were previously treated and stable. Patients with gastrointestinal impairment, high risk of developing QTc prolongation, or any other concurrent severe and/or uncontrolled medical condition that would impair the study, and patients with a known history of HIV infection were not eligible for the study.
From December 2018 until December 2022, 75 patients were enrolled in 16 Belgian sites for treatment with letrozole (2.5 mg once daily) + ribociclib 600 mg (day 1 to 21 in a 28-day cycle), which continued until disease progression, intolerable toxicity, or patient/physician decision to withdraw. During the study, patients were regularly evaluated for disease progression. Radiological examinations for response assessment were performed at 12w (+/-2w) and 24w (+/-2w). Afterwards radiological tests were further performed every 12 to 24 weeks depending on the clinical need as long as the patient was receiving treatment or during follow-up (until progression occurred). Patients were also monitored for treatment safety, for quality of life, and geriatric assessment components including functional status at 12 weeks (+/-2 weeks) and at 48 weeks (+/-2 weeks). This study was conducted in compliance with the principles of the Declaration of Helsinki, the Good Clinical Practice, and in accordance with all applicable regulatory requirements. All patients included in this study gave written informed consent before inclusion. The study protocol was approved by the ethics committee of our institution (Ethics Committee Research University Hospitals/Catholic University Leuven; study number: S61033; Belgian registration number: B322201838186; approval date: 29 November 2018).
Within the leading participating center (UH Leuven), a preplanned prospective blood biomarker study was conducted, which aimed at evaluating the impact of ribociclib and letrozole on the circulating immune profile. For this substudy, additional collection of PBMCs was implemented. The current manuscript specifically describes this biomarker study.
2.2 G8 assessment
The G8 questionnaire is a simple, widely-used geriatric screening instrument, that can be used as an estimate for frailty[24]. Eight items make up the G8: seven are taken from the mini nutritional assessment (MNA) questionnaire (nutritional status, weight loss, body mass index, motor skills, psychological status, number of medications, and self-perception of health), and one inquires the patient's age (< 80, 80 - 85, > 85). The G8-score ranges from 17 (not at all impaired) to 0 (heavily impaired). Patients with a score ≤ 14 are considered as potentially frail (increased risk of underlying geriatric problems), while those with a score of > 14 are regarded as fit[25,26].
2.3 Peripheral blood mononuclear cells (PBMCs) isolation
Peripheral blood for PBMC isolation was collected in two 10 mL EDTA tubes (BD Vacutainer®) at baseline (before ribociclib initiation) and after three months of treatment (three cycles of ribociclib + letrozole treatment; sample in principle taken after one week of interruption, just before initiation of next cycle). PBMCs were isolated within one hour after blood collection via density gradient centrifugation. In brief, an equal volume of Dulbecco's phosphate buffered saline (Invitrogen) containing 2% fetal bovine serum (Invitrogen) (DPBS/2% FBS) was added to the blood. Thereafter, two SepMate-50 tubes (StemCell Technologies) were each filled with 15 mL Histopaque®-1077 (Sigma-Aldrich). The diluted blood sample was divided over the two SepMate-50 tubes and centrifuged at 1200 xg for 10 minutes at room temperature (RT). After centrifugation, the top layer (containing the PBMCs) was poured into a 50 mL Cellstar tube (Greiner bio-one) and centrifuged again (400 xg, 10 mins, RT). After resuspension of the cell pellet, the cells were microscopically counted and assessed for viability using trypan blue (Invitrogen). Then, the cells were washed twice with DPBS/2% FBS, resuspended in 3 mL of cold freezing medium, containing 90% FBS and 10% dimethylsulfoxide (DMSO) (Sigma-Aldrich), and equally divided over three 1.8 mL cryovials (Thermo Scientific). These were frozen using a Mr. Frosty container (Thermo Scientific) filled with isopropanol (2-propanol, Merck Millipore) in a -80 °C freezer. After a minimum of four hours, PBMCs were transferred to liquid nitrogen until later use for PBMC staining.
2.4 Antibody staining and flow cytometric analysis of PBMCs
After quick thawing of the PBMCs in warm RPMI1640 medium (Invitrogen) with 10% FBS (Invitrogen), the immune subset composition profiles were analyzed using 8-color flow cytometry on a 3-laser platform (FACSVerse, BD Biosciences). The use of fluorescent antibody staining panels targeted at specific combinations of cell surface markers allowed identification and analysis of the different immune cell subsets (Figure S1 and Figure S2). In total, we used five multicolor flow cytometry staining panels to investigate changes in the immune cell subsets. Details on the specific antibodies used can be found in Table S1. Briefly, the major PBMC subsets (i.e., monocytes, NK-cells, naïve and memory B-cells, NK/T-cells, and total T-lymphocytes) were identified using the markers CD3, CD14, CD16, CD19, CD27, CD56, and HLA-DR. The CD4+ and CD8+ T-cell populations were further subtyped using the markers CD3, CD4, CD8, CD27, CD28, CD45RA, CD57, and CD197/CCR7. The costimulatory receptors CD27 and CD28, as well as the senescence marker CD57, were used as additional markers to assess age-related changes in the T-cell population. T-cell activation status was assessed with a combination of CD3, CD4, CD8, CD38, CD69, CD279 (PD-1), and HLA-DR targeting antibodies. All panels also included 7-AAD as a viability stain to allow dead cell exclusion. In addition, BD Horizon™ Brilliant Stain Buffer is added to the reagent mixtures to prevent staining artifacts and ensure optimal results with BD Biosciences dyes. The staining procedure was performed as follows: after washing the thawed PBMCs with DPBS/2% FBS, 400 μL of cells were preincubated with 20 μL FC block (Human TruStain FcX, BioLegend) for 10 minutes. Thereafter, 100 μL of the cells were added to each antibody mixture of the staining panels in FACS tubes separately and incubated for 15 minutes at RT in the dark. Then, the cells from each panel were washed twice with 2 mL of cold (4 °C) DPBS + 2% FBS and centrifuged at 500 xg, 10 mins, 4 °C. The pellet was resuspended in 400 μLcold (4 °C) DPBS+2% FBS and kept on ice in the dark until FACS analysis. Lastly, T-regulatory cells were examined via intracellular staining using the markers CD3, CD4, CD25, CD45RA, CD127, CD152 (CTLA-4), and FoxP3. The Fixable Viability Stain (FVS) 520 was used for discrimination between viable and non-viable cells. First, 100 μLof cells were stained with 1 μLof diluted FVS520 (1/100 with staining buffer, BD Pharmingen). Then, cells were washed twice with 2 mL staining buffer (BD Pharmingen), centrifuged at 400 xg for 10 minutes at RT, and resuspended. The antibody mixture was added, vortexed, and incubated for 20 minutes at RT in the dark. Cells were rewashed in 2 mL staining buffer, centrifuged at 400 xg for 10 minutes at RT, and resuspended. Thereafter, fixation and permeabilization were performed with 1x Human FoxP3 buffer (BD Pharmingen) according to the manufacturer's protocol. Then, 5 μLof conjugated FoxP3 antibody was added to resuspend and stain the cell pellet and incubated for 30 minutes in the dark at RT. Cells were washed twice with 2 mL of staining buffer and centrifuged at 500 xg for 5 minutes at RT. The cell pellet was resuspended in 300 μLstaining buffer and analyzed immediately on the FACSVerse. All the staining panels were optimized using fluorescence minus one as negative control to delineate unstained populations. Data acquisition and analysis were performed with the FACSuite software (version 1.0.6, BD Biosciences).
2.5 Plasma collection
Plasma was isolated from blood collected in a 4 mL BD Vacutainer® EDTA K2E tube at baseline (before ribociclib initiation) and after three months of treatment (three cycles of ribociclib + letrozole treatment). Within 60 minutes after blood drawing, the blood samples were centrifuged at 1600 xg for 15 minutes at 4 °C. Then, plasma was isolated and stored in aliquots at -80 °C until future use.
2.6 Analysis of plasma immune response and checkpoint markers
Plasma samples were analyzed by multiplex cytometric bead array immunoassays, using commercially available pre-mixed kits: a 13-plex, containing the analytes IL-4, IL-2, CXCL10 (IP-10), IL-1β, TNF-α, CCL2 (MCP-1), IL-17A, IL-6, IL-10, IFN-γ, IL-12p70, CXCL8 (IL-8), and free active TGF-β1 (LEGENDplex™ HU Essential Immune Response Panel, BioLegend®) and a 12-plex, containing sCD25 (IL-2RA), 4-1BB, sCD27, B7.2 (CD86), free active TGF-β1, CTLA-4, PD-L1, PD-L2, PD-1, TIM-3, LAG-3, and galactin-9 (LEGENDplex™ HU Immune Checkpoint Panel 1, BioLegend®). These immunoassays were performed according to the manufacturer's protocol on 1:2 diluted plasma samples and every sample was analyzed in duplicate. After the staining procedure, standard dilutions of known concentrations and test samples were run on a FACSVerse flow cytometer equipped with FACSuite software (version 1.0.6, BD Biosciences). After flow cytometric analysis of the standard dilutions and samples, data were analyzed with the online BioLegend LEGENDplex™ software tool (https://www.biolegend.com/en-us/legendplex).
2.7 Statistical analysis
First, the impact of the combination treatment on the immune system was investigated on the whole patient cohort, as well as within the fit (G8 > 14) and frail (G8 ≤ 14) populations separately. Paired analyses were performed using the Wilcoxon signed rank test to evaluate changes in PBMC subsets and plasma markers between baseline and after three months of combination therapy. Then, unpaired analyses were performed using the Mann-Whitney U test to evaluate differences in PBMC subsets and plasma markers between the fit (G8 > 14) and frail (G8 ≤ 14) subpopulations, both at baseline and after three months of combination treatment. Lastly, the interaction between time and frailty was analyzed as follows: first, a change score was calculated between patients' values at three months and baseline (positive values indicating increase), and second, the difference in change score was tested by the Mann-Whitney U test. This interaction test indicates whether there is a difference between fit and frail patients with respect to their evolutions over time. All tests are two-sided and evaluated at a 5% significance level. Multiple testing correction was done by applying a false discovery rate (FDR) of 0.05. Analyses have been performed using SAS software (version 9.4 of the SAS System for Windows).
3. Results
3.1 Patient characteristics
Twenty older patients with HR+/HER2- mBC were enrolled in this biomarker study at UH Leuven. All clinical and pathological characteristics can be found in Table 1. Median age was 76 years (range: 70 - 87 years). Based on the geriatric assessment, 8 patients were considered fit (G8 > 14) and 12 patients (60%) were considered frail (G8 ≤ 14). Mean age in the fit and frail subgroups was 73.5 years and 79.1 years, respectively. Invasive Breast Carcinoma of No Special Type (IBC-NST) was the most prevalent histology (70% of participants) in our study population. Bone was the most frequent site of metastasis (70%), followed by lymph nodes (55%). Primary tumor characteristics and metastatic pattern were comparable between fit and frail subgroups. Treatment dose reductions between baseline and three months were necessary in 65% of the patients (i.e. 4 out of 8 fit patients and 9 out of 12 frail patients). For these patients, doses of ribociclib were reduced to 400 mg (30%) or 200 mg (35%). Treatment had to be discontinued in 3 patients (i.e. 1 fit and 2 frail patients). Adverse events between baseline and three months were common with this treatment regimen in the older population. The most frequent grade 3 or grade 4 adverse events were neutropenia (grade 4: 10%; grade 3: 35%), hypertension (grade 3: 60%), pain (grade 3: 20%), fatigue (grade 3: 5%), and infections (grade 3: 5%) (Table S2).
Characteristics | Result | % |
Age(years) | ||
Median | 76 | |
Range | [70; 87] | |
Frailty status | ||
Fit (G8 > 14) | 8 | 40% |
Frail (G8 ≤ 14) | 12 | 60% |
ECOG-PS at baseline | ||
0 | 11 | 55% |
1 | 7 | 35% |
2 | 2 | 10% |
Histology | ||
IBC-NST | 14 | 70% |
ILC | 5 | 25% |
Mixed IBC-NST/ILC | 1 | 5% |
Tumor grade | ||
2 | 13 | 65% |
3 | 4 | 20% |
Unknown | 3 | 15% |
Disease setting | ||
Primary metastatic (de novo) | 8 | 40% |
Secondary metastatic | 12 | 60% |
Tumor biopsy for HR/HER2 determination | ||
Primary tumor | 15 | 75% |
Locoregional lymph node | 3 | 15% |
Metastasis | 2 | 10% |
Sites of metastases | ||
Bone | 14 | 70% |
Liver | 3 | 15% |
Lungs | 4 | 20% |
Pleura | 1 | 5% |
Lymph node | 11 | 55% |
Locoregional | 5 | 25% |
Other | 3 | 15% |
Number of sites with metastatic disease | ||
1 | 6 | 30% |
2 | 7 | 35% |
3 | 7 | 35% |
Dose reduction during ribociclib treatment | ||
Yes | 13 | 65% |
Dose reduction to 400 mg | 6 | 30% |
Dose reduction to 200 mg | 7 | 35% |
No | 7 | 35% |
Premature ribociclib discontinuation before three months | ||
Yes | 3 | 15% |
No | 17 | 85% |
G8: geriatric 8 health status screenings score; ECOG-PS: Eastern Cooperative Oncology Group - Performance Status; IBC-NST: invasive breast carcinoma of no special type; ILC: invasive lobular carcinoma.
3.2 Immune composition is altered upon ribociclib/letrozole treatment in the entire study cohort
To assess the impact of the combination treatment on the immune composition, we compared PBMC subset distribution profiles between baseline and three months in the whole patient population. Detailed results of the flow cytometry analyses can be found in Table S3. First, we investigated the effect of the treatment on major PBMC subsets in the entire patient cohort, including monocytes, NK-cells, B-cells, NK/T-cells, and total T-cell population. Within the B cell compartment, memory B-cells were significantly decreased (P = 0.012), whereas naïve B-cells (P = 0.012) were significantly increased after three months of letrozole + ribociclib treatment (Figure 1A). Also, a higher proportion of the monocytes exhibited the 'intermediate (CD14high CD16+)' phenotype (Figure 1B). Next, we looked deeper into specific T-cell subsets. Within the T-regulatory cells (Tregs), the naïve Treg subset had significantly expanded (P = 0.001), while the memory Treg subset had shrunken (P = 0.001) after three months of combination therapy (Figure 1C). Interestingly, the expression of CD25 on the naïve Tregs was also significantly higher after three months of treatment (P = 0.039).
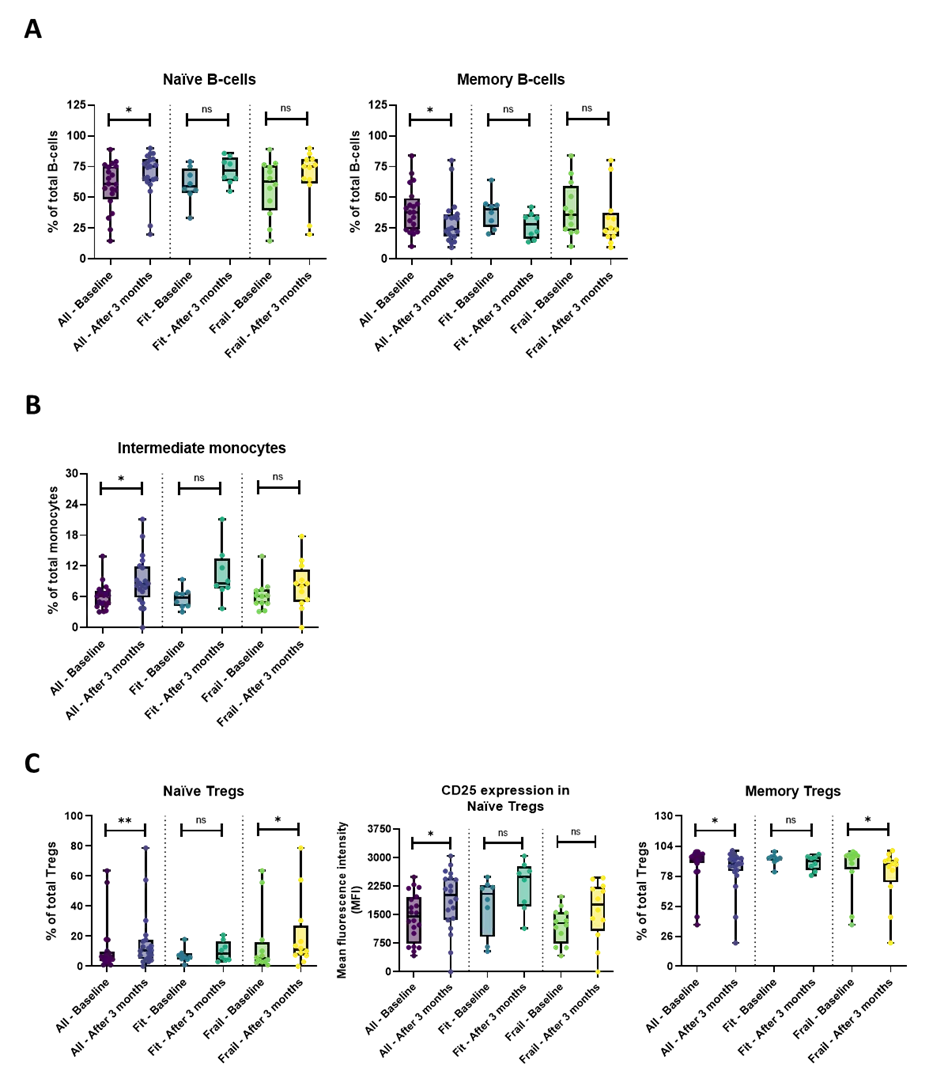
Figure 1. Overview of the B-cell, monocyte, and T-regulatory subsets in the whole patient cohort and the fit and frail patient cohort separately. (A) After three months of combination treatment, the naïve B-cells were significantly increased, while the memory B-cells were significantly decreased in the entire patient cohort. No significant results were demonstrated in the fit and frail population; (B) The proportion of intermediate monocytes was significantly higher after three months of treatment in the whole patient cohort, while within the fit and frail patient cohort, no significance was found; (C) Within the Treg population, the percentage of naïve Tregs was significantly higher, along with an increased expression of CD25. In contrast, the memory Tregs were significantly decreased after three months of combination treatment. In the frail population, the naïve Tregs were increased as well, while the memory Tregs were significantly decreased. In the fit population, no significant results were demonstrated. The boxplots represent the IQR of the percentage of the specific immune cell subsets or the MFI value. The level of significance is indicated with * for FDR-corrected P ≤ 0.05, with ** for P ≤ 0.01, and with ns: no statistical significance. CD: cluster of differentiation; Tregs: T-regulatory cells; MFI: mean fluorescence intensity; FDR: false discovery rate.
Within the CD4+ T-cell subset, numerous parameters significantly changed (also after multiple testing correction) during the treatment in the entire patient cohort. Naïve CD4+ T-cells increased (P = 0.022) together with an upregulated expression of the costimulatory receptors CD27 (P = 0.005) and CD28 (P = 0.024). In addition, the double positive naïve CD4+CD27+CD28+ T-cells were also significantly more abundant (P = 0.005) and exhibited upregulated expression of their costimulatory receptors CD27 (P = 0.010) and CD28 (P = 0.032) at three months, as compared to baseline (Figure 2A and Figure S3A). Concomitant with the expansion of the naïve subset, the frequency of central memory (CM) CD4+ T-cells was decreased (P = 0.026). Yet, similar to the naïve subset, the expression of the costimulatory receptors CD27 and CD28 was also significantly elevated (P = 0.005 and P = 0.028, respectively) on CM CD4+ T cells. Also here, the treatment appeared to promote the double positive CM CD4+CD27+CD28+ phenotype (P = 0.004), along with upregulated expression of CD27 (P = 0.005) and CD28 (P = 0.024) on these CM CD4+CD27+CD28+ T-cells (Figure 2B and Figure S3B). Although the frequency of effector memory (EM) CD4+ T-cells was not significantly altered, their expression of CD27 and CD28 did show a significant increase after three months of combination treatment (P = 0.005 and P = 0.022, respectively). Again, a significant expansion of the fully functional double positive EM CD4+CD27+CD28+ T-cell subset (P = 0.005), which moreover showed elevated expression levels of CD27 (P = 0.005) and CD28 (P = 0.007), was noticed at three months (Figure 2C and Figure S3C). Similar observations were made for the terminally differentiated effector memory re-expressing CD45RA (TEMRA) CD4+ T-cells: the treatment induced significant upregulation of the costimulatory receptors CD27 and CD28 (P = 0.008 and P = 0.022, respectively) (Figure 2D) and a higher fraction of the double positive TEMRA CD4+CD27+CD28+ T-cells, (P = 0.005) which additionally showed increased expression of CD27 (P = 0.005) and CD28 (P = 0.005) (Figure 2D and Figure S3D).
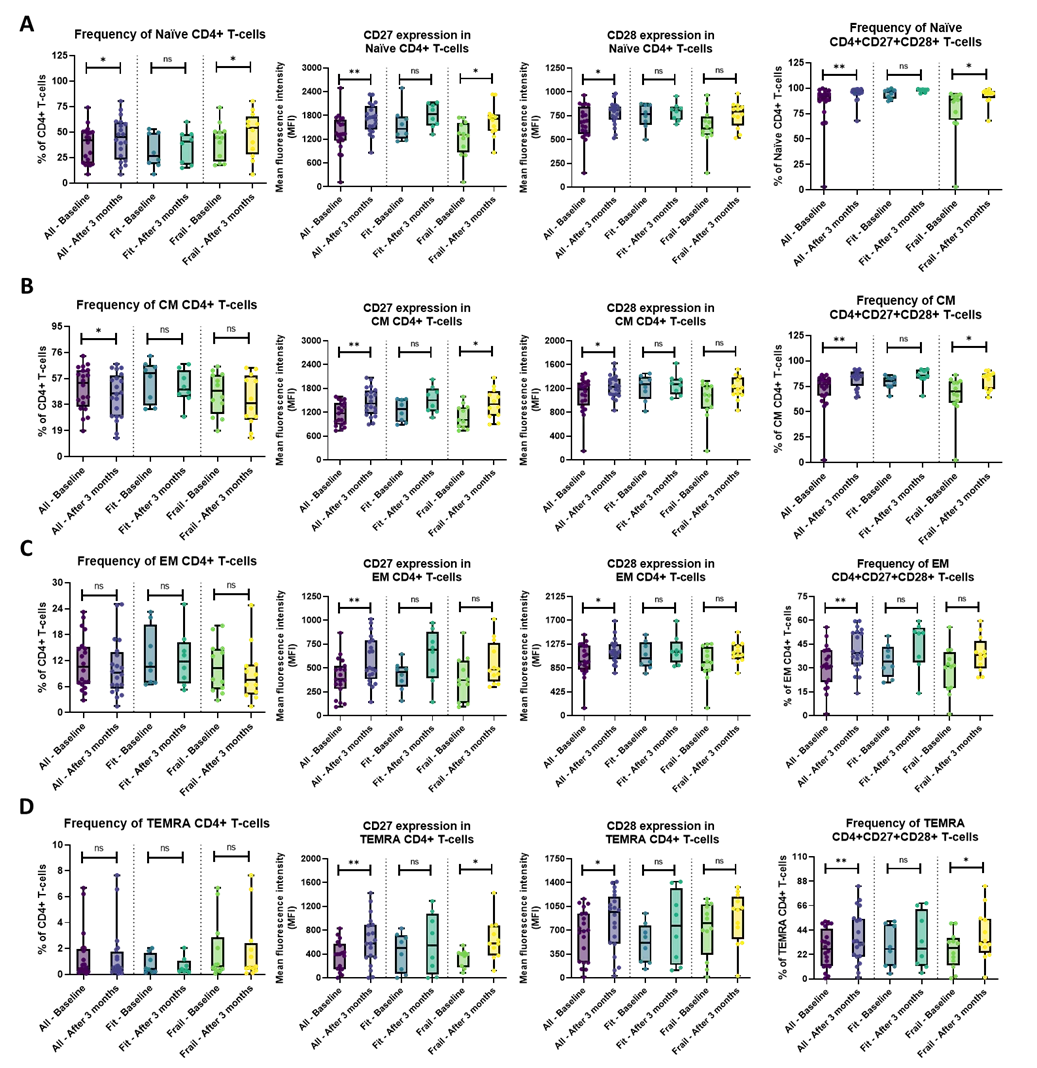
Figure 2. Overview of the CD4+ T-cell subsets in the whole patient cohort and the fit and frail patient cohort separately. (A) In the entire patient cohort, the percentage of naïve CD4+ (CD27+CD28+) T-cells was significantly higher after three months of treatment, along with a significantly increased expression of the costimulatory receptors CD27 and CD28. Within the frail patient cohort, the naïve CD4+ (CD27+CD28+) T-cells were significantly increased, along with an increased CD27 expression. No significant differences were found after three months of combination treatment in the fit patient population; (B) The percentage of CM CD4+ T-cells significantly decreased, while the CM CD4+CD27+CD28+ T-cell population and the CD27 and CD28 receptors' expression significantly increased in the whole patient cohort. In the frail cohort, only the increased expression of CD27 and CM CD4+CD27+CD28+ T-cell population after three months of treatment was seen. No significant results were demonstrated in the fit patient population; (C) In the entire patient population, after three months of treatment, the expression of CD27 and CD28 of the EM CD4+ T-cells and EM CD4+CD27+CD28+ T-cells were significantly elevated, while in the fit and frail patient population, no significance was shown; (D) The expression of CD27 and CD28 of the TEMRA CD4+ T-cells and frequency of TEMRA CD4+CD27+CD28+ T-cells was significantly increased in the whole patient cohort. Within the frail population, a significantly elevated CD27 expression and an increased frequency of TEMRA CD4+CD27+CD28+ T-cells were seen. Within the fit population, no significant results were demonstrated. The boxplots represent the IQR of the percentage of the specific CD4+ T-cell subsets or the mean fluorescence intensity (MFI) value. The level of significance is indicated with * for FDR-corrected P ≤ 0.05, with ** for P ≤ 0.01, and with ns: no statistical significance. CD: cluster of differentiation; CM: central memory; EM: effector memory; TEMRA: terminally differentiated effector memory re-expressing CD45RA.
Within the CD8+ T-cell population, the treatment-induced changes basically paralleled those observed in the CD4+ T-cells, although upregulation of costimulatory receptors was rather confined to CD27, while the intensity of CD28 staining was not significantly altered upon treatment (Table S3). For example, the CD27 expression of the CD8+ naïve T-cells was significantly increased at three months (P = 0.023). In addition, the frequency of naïve CD8+CD27+CD28+ T-cells (P = 0.023), as well as their CD27 expression level (P = 0.019), were significantly elevated (Figure 3A and Figure S4A). Similar observations were seen for the CM and EM CD8 T-cell subsets (Figure 3B,C and Figure S4B,C). No significant differences were found within the TEMRA CD8+ T-cell population (Figure 3D and Figure S4D).
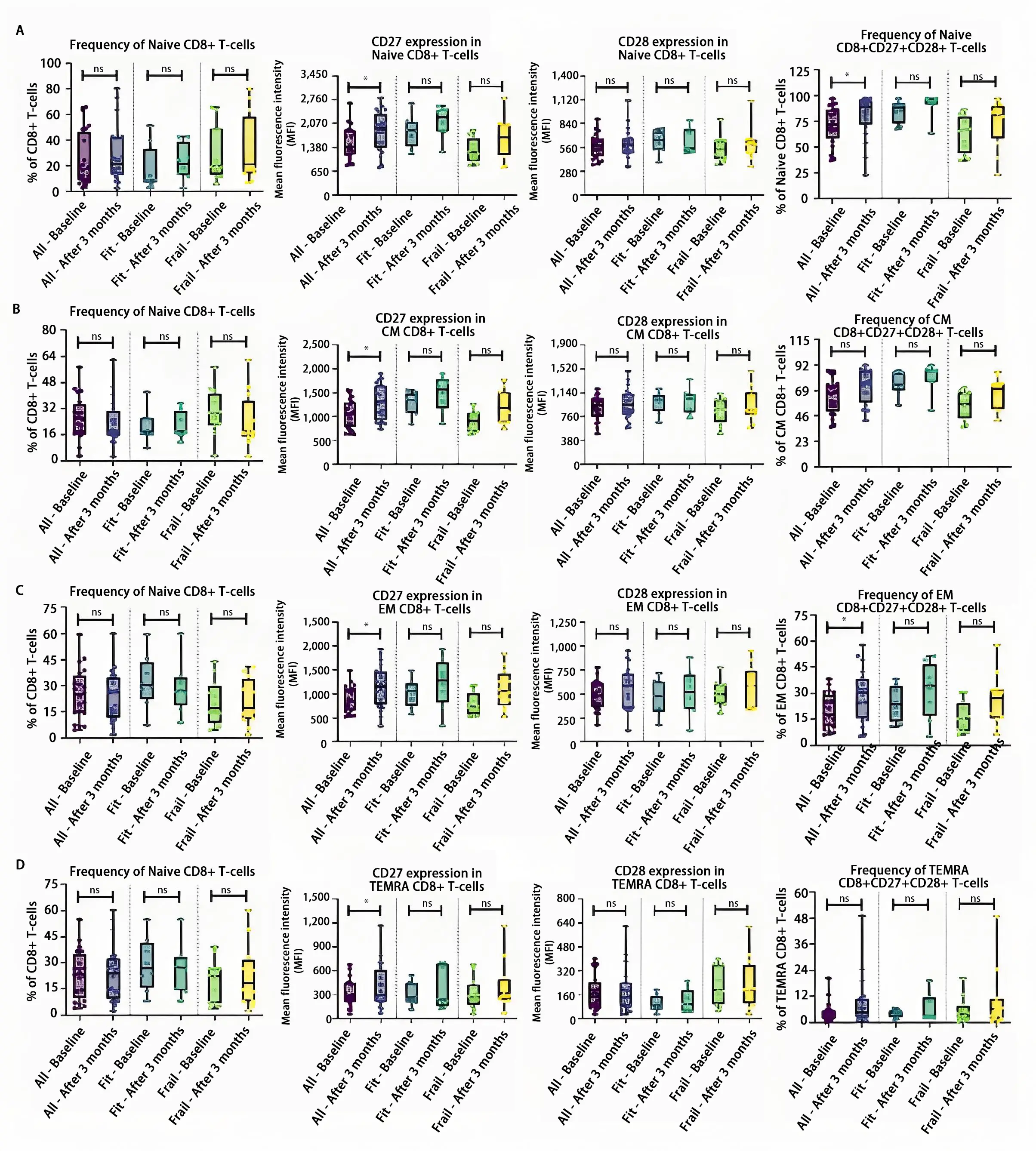
Figure 3. Overview of the CD8+ T-cell subset in the whole patient cohort and the fit and frail patient cohort separately. (A) In the entire cohort, the CD27 expression of the naïve CD8+ T-cells together with the double positive naïve CD8+CD27+CD28+ T-cells was significantly increased after three months of combination treatment, while in the fit and frail cohort, no significance was found; (B) In the CM CD8+ T-cell population, the expression of the costimulatory receptor CD27 was significantly increased in the whole patient population. After three months of treatment, no significant differences were noticed within the fit and frail population; (C) In the entire cohort, the CD27 expression of the EM CD8+ T-cells was significantly elevated together with an increase of the EM CD8+CD27+CD28+ T-cells after three months of treatment, while in the fit and frail cohort, no significant differences were found; (D) No statistically significant difference was found after three months of combination therapy in the TEMRA subpopulation within the entire cohort nor in the fit and frail cohort separately. The boxplots represent the IQR of the mean fluorescence intensity (MFI) value. The level of significance is indicated with * for FDR-corrected P ≤ 0.05 and with ns: no statistical significance. CD: cluster of differentiation; CM: central memory; EM: effector memory; TEMRA: terminally differentiated effector memory re-expressing CD45RA.
Unlike the costimulatory receptors CD27 and CD28, the expression of the activation markers CD38, CD69, HLA-DR, and CD279 (PD-1) on CD4+ and CD8+ T-cells showed no marked differences between baseline and after three months of combination treatment in the whole patient cohort after correction for multiple testing (Table S3).
Regarding the plasma immune response and checkpoint markers, only circulating B7.2 (CD86) and PD-1 were significantly reduced after three months of combination therapy (P = 0.017 and P = 0.029, respectively), when evaluated in the entire patient cohort (Figure 4A,B).
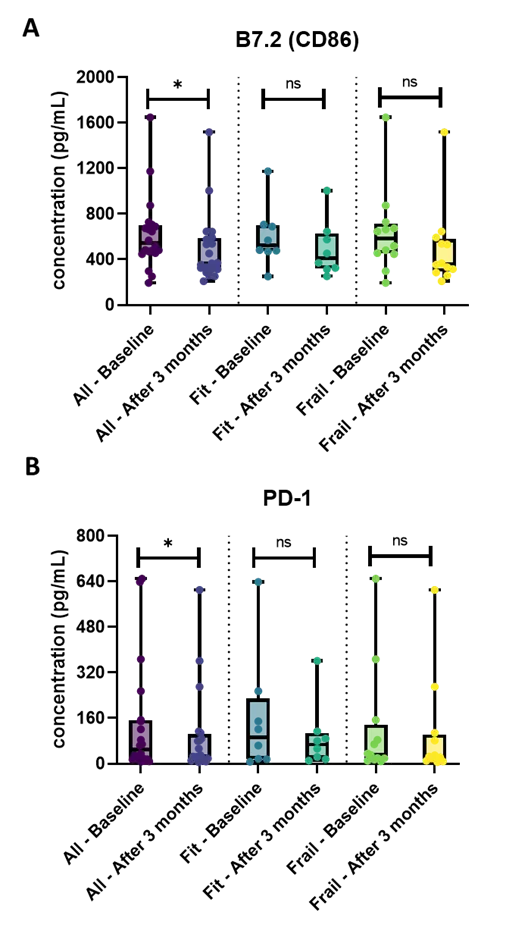
Figure 4. Overview of the plasma immune response and checkpoint markers in the whole patient cohort and the fit and frail patients cohort separately. (A) The plasma concentration of B7.2 (CD86) was significantly decreased after three months of combination treatment in the entire patient population. No significant results within the frail and fit population were demonstrated; (B) In the whole patient cohort, the concentration of plasma PD-1 was significantly lower after three months of treatment, while in the fit and frail patient cohort, no significance was found. The boxplots represent the IQR of plasma immune response and checkpoint markers' concentration (pg/mL). The level of significance is indicated with * for FDR-corrected P ≤ 0.05 and with ns: no statistical significance. FDR: false discovery rate; IQR: interquartile range; PD-1: programmed cell death protein 1.
3.3 Impact of ribociclib/letrozole treatment on the immune profile in fit and frail patients
If we consider treatment-induced changes in the fit (Table S4) and frail (Table S5) populations separately, the naïve Tregs (P = 0.019) were significantly increased and memory Tregs (P = 0.019) were significantly reduced upon treatment solely in the frail, but not in the fit subpopulation (Figure 1C).
Also, within the frail population, significant increases of naïve CD4+ T-cells (P =0.045), with upregulated CD27 expression (P = 0.038) were noted after three months of combination treatment (Figure 2A). Upregulation of CD27 was also found in the CM and TEMRA CD4+ T-cell populations (P = 0.032 for both) after three months of treatment (Figure 2B,D). Moreover, the frequency of CD27+CD28+ double positive cells was significantly increased in the naïve, CM, and TEMRA CD4+ T-cell subsets of frail patients at three months (P -values were 0.032, 0.016, and 0.032, respectively) (Figure 2A, B, D). Moreover, the expression of the costimulatory receptors seemed to be increased on these CD27+CD28+ double positive subsets upon treatment in the frail population (Figure S3). Interestingly, the fraction of activated CD8+ T-cells, expressing CD38, was also significantly expanded at three months compared to baseline in frail patients (P = 0.047) (Table S5). Conversely, in the fit population, none of the above-described changes could be demonstrated with statistical significance when applying multiple testing correction (Table S4).
To further investigate whether the evolution over time of the various immune markers was different in fit versus frail patients, we also performed an interaction analysis between time and frailty. To this end, we calculated a change score between each patient's values at three months and baseline (positive values indicating increase), and these change scores were compared between fit and frail patients. After correction for multiple testing, no significant differences were found (Table S6), indicating that in this small cohort studied here, fit and frail patients essentially did not differ with regard to their evolutions over time for any of the immune markers (PBMC subsets as well as plasma biomarkers) tested.
Lastly, when applying multiple testing correction, no significant changes in the plasma immune response and checkpoint markers could be retained, neither in fit nor in frail patients (Table S4 and Table S5).
3.4 Comparison of the immune biomarkers in fit versus frail patients at baseline or after three months of combination treatment
Next, we evaluated if there were significant differences in the immune subset composition and/or in plasma circulating immune biomarkers between fit and frail patients, either at baseline (Table S7) or after three months of ribociclib/letrozole combination treatment (Table S8). No significant alterations were found after correction for multiple testing.
4. Discussion
Endocrine therapy has been used for several decades as first-line treatment option in (older) patients with HR+/HER2- mBC. However, the efficiency of treating HR+/HER2- mBC is seriously hampered by endocrine treatment resistance[27,28]. CDK4/6 inhibitors have entered the treatment landscape, as these drugs appeared to delay endocrine resistance and to improve disease control, prolong survival, and quality of life when given in combination with ET[12-17]. Even in the understudied older population, substantial benefit is noticed when administering CDK4/6 inhibitors together with ET, as shown in the MONALEESA-2 study[13,18,19]. Apart from blocking the cell cycle, CDK4/6 inhibitors seem to exert immunomodulatory effects as well. A preclinical study by Deng et al.[21]described that CDK4/6 inhibitors promote T-cell activation, mainly due to increased T-cell recruitment and enhanced effector cell function in tumors. Furthermore, they reported that Tregs are more susceptible to CDK4/6 inhibition[21]. These findings are also confirmed by Goel et al.[16], who found that CDK4/6 inhibitors reduce the Treg population. The authors suggested that the selective Treg depletion might be linked to the fact that these cells express higher levels of Retinoblastoma 1 (Rb1), which is a key mediator of the CDK4/6 signaling pathway. Furthermore, they also point out that high abundance of regulatory T-cells in estrogen-positive BC tumors is associated with a poor clinical outcome[16]. However, little research has been done on how these CDK4/6 inhibitors would alter the immune profile of older HR+/HER2- mBC patients, where the immune microenvironment is already affected by the advancing aging process.
Our study explored the influence of the CDK4/6 inhibitor ribociclib in combination with letrozole, an aromatase inhibitor, on PBMC subset distribution and on a wide panel of plasma immune response and checkpoint markers in older (≥ 70 years) patients with HR+/HER2-mBC (Figure 5). The main findings revealed that after three months of ribociclib/letrozole combination treatment naïve CD4+ T-cells, naïve B-cells, and naïve Tregs were significantly upregulated, whereas memory B-cells and memory Tregs were significantly downregulated. In addition, within the CD4+ and CD8+ T-cell subsets, the expression of the costimulatory receptors CD27 and CD28 was significantly upregulated after three months of this treatment. Lastly, we observed that the soluble plasma immune checkpoint markers B7.2 (CD86) and PD-1 were significantly downregulated upon treatment.
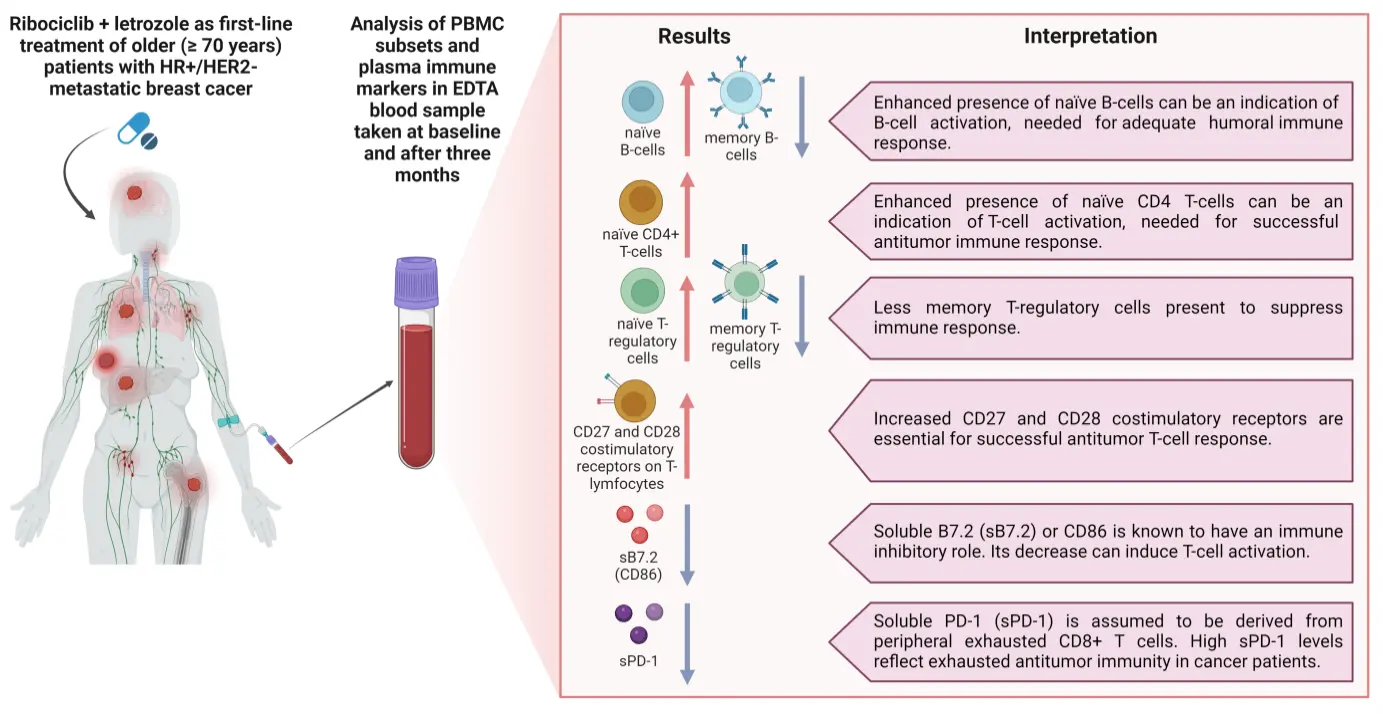
Figure 5. Immunomodulatory effect of ribociclib and letrozole in older (≥ 70 years) patients with metastatic HR+/HER2- breast cancer. Blood samples were collected at baseline and after three months of therapy and changes in PBMC subsets and plasma immune markers were assessed. Created in BioRender.com. CD: cluster of differentiation; HER2: human epidermal growth factor 2; HR: hormone receptor; PBMC: peripheral blood mononuclear cell; PD-1: programmed cell death protein 1.
As mentioned before, within the older individuals, the immune function is already altered by the age-related process of immunosenescence[3]. This mainly results in a decreased adaptive immunity, which can impact the ability of immune cells to recognize and respond adequately to a developing tumor and thus can affect the tumor's immune response. In particular, immunosenescence is characterized by a decrease of naïve cells concurrent with an accumulation of memory cells, most notably in the T-cell population[3]. Furthermore, Koch et al. described that immunosenescence results in a reduced expression of the costimulatory receptors CD27 and CD28[29]. Elyahu and colleagues reported that at old age, T-cells are more exhausted, and Treg cell frequency and functionality are elevated[30]. Also, Fulop and colleagues found that old(er) age is associated with an elevated expression of immune checkpoint markers on T-cells such as CTLA-4 and PD-1[31]. These findings all illustrate the profound remodeling that is happening in the aging immune system. However, when we reflect on our own results, we see that the treatment with CDK4/6 inhibitors and ET actually reverses the age-related immunosenescence process, with increasing naïve T-cell and B-cell populations and decreasing memory populations. In addition, the costimulatory receptors CD27 and CD28 are increased. CD27 and CD28 costimulatory receptors are essential for T-cell survival and activation by (tumor) antigens and, consequently, for successful (anti-tumor) T-cell responses[29]. Interestingly, circulating plasma B7.2 (CD86) was significantly decreased in our study cohort after three months of combination treatment. B7.2 (CD86) is a cell surface protein-ligand predominantly expressed on antigen-presenting cells (and some tumor cells) and can bind both CD28 and CTLA-4, two receptors on T-lymphocytes that regulate the immune response. The B7.2 (CD86)-CD28 ligand-receptor interaction evokes a costimulatory signal resulting in IL-2 production, which is essential for T-lymphocyte proliferation[32]. A soluble form sB7.2 (CD86), lacking the transmembrane domain, is generated by alternative splicing of the full-length B7.2 mRNA transcript[33]. Although the biological role of sB7.2 (CD86) is not yet fully understood, it was suggested that the release of sB7.2 (CD86) affords a powerful mechanism by which cells may modulate the costimulatory signals delivered through membrane-bound B7.2 (CD86)[33]. Several studies have reported increased levels of sB7.2 (CD86) in cancer. An immune inhibitory role of sB7.2 (CD86) was suggested via binding to CTLA-4 and inducing negative signaling to T-lymphocytes. On the other hand, an inhibitory feedback role of sB7.2 (CD86) on the costimulatory CD28 signaling induced by membrane-bound B7.2 (CD86) has also been proposed[33]. This hypothesis is in agreement with our findings that circulating plasma B7.2 (CD86) is diminished concurrent with an expansion of the naïve CD4+ T-cell population after three months of ribociclib/letrozole combination therapy. This points to higher proliferation within the CD4+ T-cell pool, which could be ascribed to reinforcement of costimulatory CD28 signaling due to lower sB7.2 (CD86) levels and thus waning of the negative feedback loop. Our data however did not show an increase of circulating plasma IL-2 levels.
Besides sB7.2 (CD86), plasma circulating soluble PD-1 was also significantly decreased after three months of combination treatment. PD-1 is part of the immune checkpoint pathway PD-1/PD-L1 which dampens T-cell activity after an antigen-induced immune response, in order to avoid sustained immune activation[32,34]. In a cancer setting, PD-1 ligation to its ligands PD-L1 and PD-L2 expressed on tumor cells or antigen-presenting cells, results in T-cell inhibition and immune escape. Blockade of the PD-1/PD-L1 axis with monoclonal antibodies (immune checkpoint inhibitors such as pembrolizumab or atezolizumab) have revolutionized the therapy of several cancer types[33,35,36]. Similar to sB7.2 (CD86), soluble variants of both PD-1 (sPD-1) and its ligands (sPD-L1, sPD-L2) are produced by alternative splicing. These can be detected in the plasma of healthy individuals, and elevated levels are found in autoimmune diseases, chronic infections, and various cancers, with increased levels being associated with worse outcomes in cancer patients[33,36]. Furthermore, ribociclib plus letrozole treatment for three months resulted in an upregulation of the Tregs naïve subset. This could possibly indicate that naïve Tregs are less activated and thus exert less of their immunosuppressive effect (which is compatible with immune stimulation). This could explain the observed accumulation of naïve Tregs and concomitant reduction of the memory Treg subset in our older patient cohort after three months of treatment. Notably, these observations confirm recent findings reported by Scirocchi et al.[37], that the total Tregs and specifically the effector Treg subset was significantly reduced during treatment with CDK4/6 inhibitors. Nonetheless, our results could not demonstrate a statistically significant decrease in the total Treg population. Taken together, these findings strengthen the evidence that CDK4/6 inhibitors provoke an immunomodulatory effect and stimulate immune function as an additional mode of action, even in the aged BC population, where immunosenescence becomes an important determinant in the patient's immune landscape.
As already mentioned, older patients (both fit and frail) represent a major part of the HR+/HER2- BC population. CDK4/6 inhibitors appear to be beneficial in older people in general, but it is a well-known phenomenon that pivotal studies in cancer do not include frail older people, although those make up a major part of the older population[19]. As a result, safety profiles, and optimal dosing of anticancer drugs in the frail subgroup is not well documented[9,19,38]. It is thus critical to assess the effectiveness and tolerability of CDK4/6 inhibitors in older BC cohorts, including both fit and frail individuals. We found no statistically significant difference in any of the immune parameters tested when comparing fit and frail patients within our study cohort, neither at baseline nor at three months. In addition, the evolution in PBMC subset composition and plasma biomarkers between the two time points did not differ between fit and frail patients, as assessed by frailty*time interaction analysis with correction for multiple testing. These findings indicate that the frailty status of an older BC patient has no impact on the immune-related effects of this treatment.
This study has some limitations, most importantly the small sample size (n = 20) with a relative dominance of frail patients in the cohort (12 frail compared to 8 fit patients). This could explain why the changes in immune markers observed in the frail subgroup were not significant in the fit subgroup. The subanalyses comparing fit and frail patients should thus be interpreted very cautiously and larger studies are needed to clearly dissect differential effects according to fitness/frailty status. In addition, this translational study was conducted in one single center; for feasibility reasons, PBMCs for translational endpoints of the RibOB trial were only collected from patients enrolled at the University Hospital in Leuven, Belgium. This may lead to patient selection bias, necessitating further research and validation in larger independent cohorts in multicentric prospective trials with an even distribution of fit and frail older patients. Another limitation is that we did not have access to a healthy control group without cancer and not treated with letrozole and CDK4/6 inhibitor; we could thus not monitor spontaneous fluctuations of the different PBMC subsets over time and/or with seasonal changes. Furthermore, the impact of the CDK4/6 inhibitor ribociclib in combination with ET on the immune system was only examined at two time points, i.e., baseline and after three months of treatment. In this way, it was not possible to dissect the exact dynamics of the immunomodulatory effects during treatment. Collecting PBMC and plasma samples at multiple time points in large prospective studies could give us a better insight into the immune-related effects of the combination treatment, both in the short and the long term. Furthermore, only a selection of specific PBMC subsets and plasma immune markers were evaluated to create a global picture of the immunomodulatory changes of the combination treatment. For instance, we did not include a PBMC staining panel for myeloid derived suppressor cells (MDSCs), because the currently used blood collection and processing procedure, including PBMC freezing, did not allow subsequent staining of MDSCs, which is only possible when working with freshly isolated PBMC. Within this study, we still need to evaluate a possible association between the change in the immune composition and therapy response in terms of progression-free survival (PFS) because follow-up is too short. With an expected median PFS of 24 months on first-line aromatase inhibitor plus CDK4/6 inhibitor, we plan to do this analysis in the upcoming year. Also, RibOB used simultaneous administration of CDK4/6 inhibitor ribociclib and letrozole as 'standard' first-line therapy in the older population. Hence, we cannot distinguish the contributions of either component to the immune-related effects of the combination treatment, especially in view of the versatile crosstalk between the endocrine system and immunity, which becomes even more complex in the context of aging[39,40]. The recently presented SONIA trial[41] suggests that it may be safe to use an aromatase inhibitor alone as first-line monotherapy, and only add the CDK4/6 inhibitors (to fulvestrant) in second line. We assume that the results would be comparable when CDK4/6 inhibitors would be used in second line, but this also requires further confirmation. Lastly, we did not assess the immunomodulatory effects of the other CDK4/6 inhibitors palbociclib and abemaciclib. As a result, we are unable to conclude whether or not the observed effects on host immunity are universal to CDK4/6 inhibition in general.
Nevertheless, this study has also major strengths. The study cohort was prospectively collected from a homogenous group of metastatic HR+/HER2- BC patients. Most importantly, this research has never been conducted before in the older HR/HER2- mBC population in order to understand what is happening with the immune system when older/clinically frail patients, most likely exhibiting some degree of immunosenescence, are treated for mBC with this standard treatment regimen. This study could be a landmark in the geriatric oncology field, as too few studies are conducted in the older BC population, including both fit and frail patients.
5. Conclusion
For the first time, we investigated whether CDK4/6 inhibitors in combination with ET affect the immune system in older HR+/HER2- mBC patients. Our results suggest that the CDK4/6 inhibitor ribociclib influences the anticancer immune response by shifting the balance from immunosuppression to immunological activation. Furthermore, CDK4/6 inhibitors combined with ET exerted a comparable immunomodulatory impact on fit and frail patients. However, to further validate these findings, there is a need for more extensive independent prospective studies involving larger cohorts of older BC patients (including fit and frail patients). In addition, apart from PBMC subsets and plasma immune markers, TCR sequencing analyses on PBMC could be an interesting technique to generate a more comprehensive picture of the dynamic changes in the immune environment during treatment.
Supplementary materials
The supplementary material for this article is available at: Supplementary materials.
Acknowledgements
We would like to thank Razgar Seyed Rahmani and prof. Diether Lambrechts (KU Leuven-VIB, Belgium) for their collaboration on this project and their valuable input in data interpretation.
Authors contribution
Wildiers H: Conceptualization, funding acquisition, methodology, project administration, resource, supervision, writing original draft.
Hatse S: Conceptualization, investigation, methodology, project administration, supervision, writing original draft.
Kenis C: Conceptualization, methodology, project administration, resource.
Neven P: Conceptualization, methodology, resource.
Lambrechts Y: Data curation, funding acquisition, investigation, methodology, project administration, visualization, writing original draft.
Decoster L, de Azambuja: Methodology, resource.
Quaghebeur C, Cornelis F, Verhoeven D, Barbeaux A, Mebis J, Van Dievel J, Verschaeve V, Duck L, De Cuypere E: Resource.
Floris G: Writing original draft.
Laenen A: Formal analysis.
Pondé N: Methodology.
All authors participated in writing, review & editing.
Conflicts of interest
Hans Wildiers: his institution received financial compensation on his behalf for advisory boards, lecture fees and/or consultancy fees from Daiichi Sankyo, Gilead, Lilly, Pfizer, Novartis, MSD, Relay Therapeutics, PSI, Augustine Therapeutics, Astra Zeneca, Roche. He received travel support from Gilead, Daiichi Sankyo, Pfizer.
Sigrid Hatse is an Editorial Board member of Ageing and Cancer Research & Treatment.
Ethical approval
The study protocol was approved by the ethics committee of our institution (Ethics Committee Research University Hospitals/Catholic University Leuven; study number: S61033; Belgian registration number: B322201838186; approval date: 29 November 2018).
Consent to participate
All patients included in this study gave written informed consent before inclusion.
Consent for publication
Not applicable.
Availability of data and materials
The data and materials could be obtained from the corresponding author.
Funding
This work is supported by the Emmanuel Van der Schueren funding from Kom op Tegen Kanker and an unrestricted research grant from Novartis.
Copyright
© The Author(s) 2024.
References
-
1. Derhovanessian E, Solana R, Larbi A, Pawelec G. Immunity, ageing and cancer. Immun Ageing. 2008;5:1-16.
[DOI] -
2. López-Otín C, Blasco MA, Partridge L, Serrano M, Kroemer G. The hallmarks of aging. Cell. 2013;153(6):1194-1217.
[DOI] -
3. Berben L, Floris G, Wildiers H, Hatse S. Cancer and aging: two tightly interconnected biological processes. Cancers. 2021;13(6):1400.
[DOI] -
4. López-Otín C, Blasco MA, Partridge L, Serrano M, Kroemer G. Hallmarks of aging: An expanding universe. Cell. 2023;186(2):243-278.
[DOI] -
5. White MC, Holman DM, Boehm JE, Peipins LA, Grossman M, Henley SJ. Age and cancer risk: a potentially modifiable relationship. Am J Prev Med. 2014;46(3):S7-S15.
[DOI] -
6. Hanahan D, Weinberg RA. Hallmarks of cancer: the next generation. Cell. 2011;144(5):646-674.
[DOI] -
7. Bailur J, Pawelec G, Hatse S, Brouwers B, Smeets A, Neven P, et al. Immune profiles of elderly breast cancer patients are altered by chemotherapy and relate to clinical frailty. Breast Cancer Res. 2017;19:1-10.
[DOI] -
8. Freedman RA, Vaz-Luis I, Barry WT, Lii H, Lin NU, Winer EP, et al. Patterns of chemotherapy, toxicity, and short-term outcomes for older women receiving adjuvant trastuzumab-based therapy. Breast Cancer Res Treat. 2014;145:491-501.
[DOI] -
9. Jenkins EO, Deal AM, Anders CK, Prat A, Perou CM, Carey LA, et al. Age-specific changes in intrinsic breast cancer subtypes: a focus on older women. The Oncologist. 2014;19(10):1076-1083.
[DOI] -
10. Argyriou AA, Polychronopoulos P, Koutras A, Iconomou G, Gourzis P, Assimakopoulos K, et al. Is advanced age associated with increased incidence and severity of chemotherapy-induced peripheral neuropathy? Support Care Cancer. 2006;14:223-229.
[DOI] -
11. Wildiers H, Kunkler I, Biganzoli L, Fracheboud J, Vlastos G, Bernard-Marty C, et al. Management of breast cancer in elderly individuals: recommendations of the International Society of Geriatric Oncology. Lancet Oncol. 2007;8(12):1101-1115.
[DOI] -
12. Hortobagyi GN, Stemmer SM, Burris HA, Yap YS, Sonke GS, Paluch-Shimon S, et al. Ribociclib as first-line therapy for HR-positive, advanced breast cancer. N Engl J Med. 2016;375(18):1738-1748.
[DOI] -
13. Hortobagyi GN, Stemmer SM, Burris HA, Yap YS, Sonke GS, Paluch-Shimon S, et al. Updated results from MONALEESA-2, a phase III trial of first-line ribociclib plus letrozole versus placebo plus letrozole in hormone receptor-positive, HER2-negative advanced breast cancer. Ann Oncol. 2018;29(7):1541-1547.
[DOI] -
14. Tripathy D, Im SA, Colleoni M, Franke F, Bardia A, Harbeck N, et al. Ribociclib plus endocrine therapy for premenopausal women with hormone-receptor-positive, advanced breast cancer (MONALEESA-7): a randomised phase 3 trial. Lancet Oncol. 2018;19(7):904-915.
[DOI] -
15. Goetz MP, Toi M, Campone M, Sohn J, Paluch-Shimon S, Huober J, et al. MONARCH 3: abemaciclib as initial therapy for advanced breast cancer. J Clin Oncol. 2017;35(32):3638-3646.
[DOI] -
16. Goel S, DeCristo MJ, Watt AC, BrinJones H, Sceneay J, Li BB, et al. CDK4/6 inhibition triggers anti-tumour immunity. Nature. 2017;548(7668):471-475.
[DOI] -
17. Braal CL, Jongbloed EM, Wilting SM, Mathijssen RHJ, Koolen SLW, Jager A, et al. Inhibiting CDK4/6 in breast cancer with palbociclib, ribociclib, and abemaciclib: similarities and differences. Drugs. 2021;81:317-331.
[DOI] -
18. Sonke GS, Hart LL, Campone M, Erdkamp F, Janni W, Verma S, et al. Ribociclib with letrozole vs letrozole alone in elderly patients with hormone receptor-positive, HER2-negative breast cancer in the randomized MONALEESA-2 trial. Breast Cancer Res Treat. 2018;167:659-669.
[DOI] -
19. Battisti NML, De Glas, Sedrak MS, Loh KP, Liposits G, Soto-Perez-de-Celis E, et al. Use of cyclin-dependent kinase 4/6 (CDK4/6) inhibitors in older patients with ER-positive HER2-negative breast cancer: Young International Society of Geriatric Oncology review paper. Ther Adv Med Oncol. 2018;10:1758835918809610.
[DOI] -
20. Howie LJ, Singh H, Bloomquist E, Wedam S, Amiri-Kordestani L, Tang SH, et al. Outcomes of older women with hormone receptor-positive, human epidermal growth factor receptor-negative metastatic breast cancer treated with a CDK4/6 inhibitor and an aromatase inhibitor: An FDA pooled analysis. J Clin Oncol. 2019;37(36):3475-3483.
[DOI] -
21. Deng J, Wang ES, Jenkins RW, Li S, Dries R, Yates K, et al. CDK4/6 inhibition augments antitumor immunity by enhancing T-cell activation. Cancer Discov. 2018;8(2):216-233.
[DOI] -
22. Berben L, Floris G, Kenis C, Dalmasso B, Smeets A, Vos H, et al. Age-related remodelling of the blood immunological portrait and the local tumor immune response in patients with luminal breast cancer. Clin Transl Immunol. 2020;9(10):e1184.
[DOI] -
23. Wolff AC, Hammond MEH, Allison KH, Harvey BE, Mangu PB, Bartlett JMS, et al. Human epidermal growth factor receptor 2 testing in breast cancer: American Society of Clinical Oncology/College of American Pathologists clinical practice guideline focused update. J Clin Oncol. 2018;36(20):2105-2122.
[DOI] [PubMed] -
24. Decoster L, Van Puyvelde, Mohile S, Wedding U, Basso U, Colloca G, et al. Screening tools for multidimensional health problems warranting a geriatric assessment in older cancer patients: an update on SIOG recommendations. Ann Oncol. 2015;26(2):288-300.
[DOI] -
25. Kenis C, Decoster L, Van Puyvelde K, De Grève J, Conings G, Milisen K, et al. Performance of two geriatric screening tools in older patients with cancer. J Clin Oncol. 2014;32(1):19-26.
[DOI] -
26. Bellera C, Rainfray M, Mathoulin-Pélissier S, Mertens C, Delva F, Fonck M, et al. Screening older cancer patients: first evaluation of the G-8 geriatric screening tool. Ann Oncol. 2012;23(8):2166-2172.
[DOI] -
27. Saatci O, Huynh-Dam KT, Sahin O. Endocrine resistance in breast cancer: from molecular mechanisms to therapeutic strategies. J Mol Med. 2021;99(12):1691-1710.
[DOI] -
28. Hanker AB, Sudhan DR, Arteaga CL. Overcoming endocrine resistance in breast cancer. Cancer cell. 2020;37(4):496-513.
[DOI] -
29. Koch S, Larbi A, Derhovanessian E, Özcelik D, Naumova E, Pawelec G. Multiparameter flow cytometric analysis of CD4 and CD8 T cell subsets in young and old people. Immun Ageing. 2008;5:1-12.
[DOI] -
30. Elyahu Y, Hekselman I, Eizenberg-Magar I, Berner O, Strominger I, Schiller M, et al. Aging promotes reorganization of the CD4 T cell landscape toward extreme regulatory and effector phenotypes. Sci Adv. 2019;5(8):eaaw8330.
[DOI] -
31. Fulop T, Larbi A, Dupuis G, Le Page A, Frost EH, Cohen AA, et al. Immunosenescence and inflamm-aging as two sides of the same coin: friends or foes? Front Immunol. 2018;8:328099.
[DOI] -
32. Chen L, Flies DB. Molecular mechanisms of T cell co-stimulation and co-inhibition. Nat Rev Immunol. 2013;13(4):227-242.
[DOI] -
33. Khan M, Arooj S, Wang H. Soluble B7-CD28 family inhibitory immune checkpoint proteins and anti-cancer immunotherapy. Front Immunol. 2021;12:651634.
[DOI] -
34. Oner G, Altintas S, Canturk Z, Tjalma W, Berckelaer CV, Broeckx G, et al. The immunologic aspects in hormone receptor positive breast cancer. Cancer Treat Res Commun. 2020;25:100207.
[DOI] -
35. Criscitiello C, Curigliano G. Immunotherapy of breast cancer. In: Michielin O, Coukos G, editors. Immuno-Oncology. Basel: S. Karger AG; 2015. p. 30-43.
[DOI] -
36. Bailly C, Thuru X, Quesnel B. Soluble programmed death ligand-1 (sPD-L1): a pool of circulating proteins implicated in health and diseases. Cancers. 2021;13(12):3034.
[DOI] -
37. Scirocchi F, Scagnoli S, Botticelli A, Filippo AD, Napoletano C, Zizzari IG, et al. Immune effects of CDK4/6 inhibitors in patients with HR+/HER2- metastatic breast cancer: relief from immunosuppression is associated with clinical response. eBioMedicine. 2022;79:104010.
[DOI] -
38. Wildiers H, Highley MS, de Bruijn EA, van Oosterom AT. Pharmacology of anticancer drugs in the elderly population. Clin Pharmacokinet. 2003;42:1213-1242.
[DOI] -
39. Huang H, Zhou J, Chen H, Li J, Zhang C, Jiang X, et al. The immunomodulatory effects of endocrine therapy in breast cancer. J Exp Clin Cancer Res. 2021;40:1-16.
[DOI] -
40. Sellegounder D, Ferrucci L, Anbazhagan R, Basisty N. Molecular crosstalk between endocrine factors, paracrine signals, and the immune system during aging. Front Endocrinol. 2023;14:1203755.
[DOI] -
41. Sonke GS, Van Ommen-Nijhof A, Wortelboer N, van der Noot V, Swinkels ACP, Blommestein HM, et al. Primary outcome analysis of the phase 3 SONIA trial (BOOG 2017-03) on selecting the optimal position of cyclin-dependent kinases 4 and 6 (CDK4/6) inhibitors for patients with hormone receptor-positive (HR+), HER2-negative (HER2-) advanced breast cancer (ABC). J Clin Oncol. 2023;41(17_suppl):LBA1000.
[DOI]
Copyright
© The Author(s). This is an Open Access article licensed under a Creative Commons Attribution 4.0 International License (https://creativecommons.org/licenses/by/4.0/), which permits unrestricted use, sharing, adaptation, distribution and reproduction in any medium or format, for any purpose, even commercially, as long as you give appropriate credit to the original author(s) and the source, provide a link to the Creative Commons license, and indicate if changes were made.
Publisher's Note
Share And Cite