Abstract
This research aims to determine the feasibility of converting a Grade II* listed building, built in 1667 and located within a conservation area in the UK, into an eco-home by reducing energy consumption and carbon emissions. The project explores the feasibility of facilitating a change of use from a commercial building into a residential dwelling while significantly improving the energy performance. A baseline energy performance assessment is carried out using a combination of infrared thermography, monitoring of hygrothermal conditions, and thermal modelling. Different retrofitting options are applied, and the results are compared to the standard benchmarks. Hygrothermal conditions are compared to the recommended comfort level temperatures as defined in Chartered Institution of Building Services Engineers (CIBSE) Guide A. The retrofitting options showed that the building could be modified to a level that shows a performance that is more favourable than the benchmarks in two out of the three benchmark measurements. Suggested improvements are sensitive to the status of the building, location, hygrothermal behaviour, and comfort level.
Keywords
1. Background
This project explores the feasibility of converting a Grade II* listed building (a UK building of special architectural or historic interest that warrants every effort to preserve it) into an energy-efficient eco-home. There are a variety of motivating factors to do that. It has been clear for some time that there is a housing crisis in the UK, fuelled by an increase in house prices and failure to build new homes amongst other issues[1]. Therefore, facilitating a change of use of buildings that were constructed for non-residential purposes and converting these into homes may offer a solution to the housing crisis. From a cultural point of view, historic buildings must be saved, after all they are all completely unique, and the listed status aims to highlight the importance of the preservation of this type of building. It is evident that repurposing and renovating historic buildings for residential use could bring a vast array of benefits. Energy performance and the lifecycle of the building must also be analysed. For instance, it may be possible to retrofit a building, yet if the building is nearing the end of its lifecycle and hence will be demolished in the near future, retrofitting may yield little benefit. Modern homes are more energy efficient compared to their historic counterparts. According to the Home Builders Federation, at least 8 in 10 new build homes have an energy performance rating of A or B, which are the top two ratings for energy efficiency, this compares with 2.2% of existing homes[2]. This improved energy performance of new buildings, results in cost savings by the owner, as well as reduced carbon emissions on a larger scale, due to a reduction in energy use for heating purposes. Although new build homes are typically more energy efficient, there are numerous concerns with new build homes in addition to the carbon costs associated with construction. When considering the lifecycle of a new building, it may seem concerning that new build homes are only guaranteed for a period of ten years[3]. If a new home is only guaranteed for ten years, how long is it expected to last? This is somewhat controversial and depends on lots of factors but is influenced by the materials used and the maintenance of the building, with the lifecycle of buildings (typically non-residential) usually based on an operational time of fifty years[4]. There is concern with regards to the longevity and build quality of new build homes. According to an article published by the Guardian newspaper in 2017, over half of new build home owners have experienced major problems with their homes, drawing on evidence of Bovis homes paying seven million pounds in compensation as a result of building inadequate homes[5].
The paper 'Life cycle assessment and historic buildings: energy-efficiency refurbishment versus new construction in Norway' provides an extremely useful insight into the comparison of retrofitting of historic buildings compared to the construction of new buildings, for the use of residential purposes[6]. This paper documents research in the form of a life cycle assessment comparing net climate benefits of refurbishing a residential building which was constructed in the 1930s and comparing these results with the benefits of constructing a new building conforming to present building codes[6]. The results from this study showed the refurbishment of the historic building being favourable in terms of climate change mitigation for a period of 60 years, when compared to new residential construction[6].
Research suggests that new homes are more efficient, yet they may have a shorter lifespan than older homes. Hence there is an opportunity to bridge this gap by making older buildings more energy efficient, prolonging their lifespan, as opposed to building new homes which ultimately generates more carbon emissions. Furthermore, what is even more damaging is the demolition of old buildings to construct new ones, it can be said that the construction processes generate the greatest emissions over the lifecycle of a building[7].
Energy performance is becoming an even more pressing issue due to climate change and an ever-increasing population. This issue is a global problem. Shockingly, the building sector is responsible for 40% of global energy consumption, in addition to 33% of global greenhouse gas emissions[8]. This is an issue that needs to be addressed to ensure the longevity of our planet and quality of life. It is clear from research that the retrofitting of buildings can offer a solution to these problems, however the issue becomes more complex when considering the retrofitting of historic buildings. There are the aforementioned issues regarding listing status, coupled with other problems surrounding hygrothermal behaviour in old buildings, and the use of non-standard materials.
In order to determine the extent to which the case study could be converted into an eco-energy efficient home, the building must be assessed in its current state in terms of energy performance. This is undertaken via hygrothermal monitoring, thermal imaging inspection and environmental modelling using the software, Integrated Environmental Solutions Virtual Environment (IESVE). A baseline model is created using IESVE to determine the current energy and carbon outputs for the building in its current state. Different improvements are made to the building to reduce the energy output and carbon emissions. A final design is selected, and this can be compared to baseline emissions set by Chartered Institution of Building Services Engineer (CIBSE), in the CIBSE TM46 energy benchmarks publication. Additionally, the final design will be compared to the energy benchmarks set out for homes by the Department of Energy and Climate Change (DECC). Finally, the design can also be compared to the baseline, to examine the improvements in energy and carbon emissions. The baseline comparison will provide an indication to whether the building can be improved enough to meet or even exceed these baselines, and hence be classified as an eco-home.
2. Study Area
The case study used is the Old Grammar School (Grade II* listed), situated in the conservation area of Upholland, Lancashire in the UK. The building was constructed in the early 17th Century from sandstone for the purpose of a Grammar School, however it is currently a workshop[9].
The building is generally in poor condition, has been used as a workshop most recently, and has not been maintained for a number of years (Figure 1). The windows do not open, and the building is heated by four open fires. The building is constructed from sandstone and timber, with an obvious lack of insulation. Hence, the building doesn't have typical hygrothermal properties, so these have been obtained from another building that has the same size and construction. The sandstone blocks are weathered and need repointing. Data was collected via a hygrometer and through the use of a thermal imaging camera. These results were then used to calibrate a model using IESVE software. The Old Grammar School in its current state is shown in Figure 1 below.
3. Methodology
Different approaches have been followed for the purpose of the investigation: thermal imaging and hygrothermal assessment were used to investigate building envelope thermal behaviour. Environmental modelling has been used to assess the impact of building insulation on carbon and energy reduction. The approaches provide informative details regarding the existing and improved comfort level.
3.1 Thermal imaging
A walk-through assessment of the building using an infrared thermal imaging camera was carried out. This was conducted using a 'FLIR B50' camera, primarily for the purpose of identifying areas of significant heat loss. Additionally, this method can show historic alterations that have been made to the building, which is useful in older buildings, where records may not have been kept or may be limited. The whole building was scanned using the infrared camera and images were then captured of specific areas of the building that show heat loss and alterations.
3.2 Hygrothermal assessment
An assessment was carried out to determine the hygrothermal performance of the building. This was undertaken using an 'EasyLog temperature and relative humidity sensors and data logger'. Hygrothermal monitoring was undertaken at different intervals, as the results can be affected by seasonal variations in temperature. Data acquisition was divided using the ground floor and first floor. Data was obtained for a four-week period for each floor, with a 2-week interval in-between, during this time the other floor was monitored. Data was acquired on the ground floor from 23rd April until 7th May, and then again from 2nd June until 25th June. With regards to the first floor, data logs were taken from 7th May until 21st May, and from 15th June until 29th June (Table 1). This means that data was obtained for a period of 8 weeks in total, over the two main rooms of the building, given that there are no partitions, the building is just segregated by the floor and ceiling in-between the ground and first floor.
Area Subject to Monitoring | Dates | |
Set 1 | Ground Floor | 23rd April - 7th May 2021 |
First Floor | 7th May - 21st May 2021 | |
Set 2 | Ground Floor | 2nd June - 15th June 2021 |
First Floor | 15th June - 29th June 2021 |
Data acquisition began during the spring, in April when the weather was mild; these results can then be compared to the results obtained during the warmer months of May and June, to assess the fabric condition. As a result of this, data is segregated into set 1, taken at the earlier dates, and set 2, taken later when outside temperatures increased in the warmer months. The data acquisition schedule is shown in Table 1 below. The data obtained was used to calibrate the model in IESVE.
3.3 Environmental modelling
Environmental modelling was undertaken using the software IESVE. This modelling was undertaken primarily to undertake an assessment of the building in its current state, known as the baseline model. This model was then used to make changes to the building by implementing different technologies to improve the energy performance of the building and comparing this to the baseline. These models can also be compared to one another to determine the optimal retrofitting solution for this case study.
As the building is classed as a commercial building and has not been maintained in recent years, there is no domestic hot water, lighting, or auxiliary ventilation, all of which will be required if the building is to be converted into a home. Currently, the only form of heating in the building is in the form of four open fires, and as a result, an additional heating system must be installed as part of the conversion into a residential building.
To ensure the consistency of environmental conditions and since the property is not in use, the thermal models considered Liverpool John Lennon weather station (UK) for purpose of the simulation. The weather file contains 12 typical meteorological months composed of hourly weather data records. Datasets with the purpose of predicting the average heating. Figure 2 shows the average monthly outdoor air dry-bulb temperatures along with relative humidity (RH). It is evident that the studied site experiences temperature fluctuations that range from 16 ℃ in summer to 5 ℃ in winter, while RH ranges from 75% to 98%.
All this information is reflected in the model, data has been inputted as true to the building as possible. As a result of this, the baseline model will provide an accurate view of the building in its current state, yet the results generated by the model in terms of energy output and carbon emissions are considered to show an underestimation and not an accurate picture compared to using the building for residential use. This is simply because the building is not equipped with the necessary features required in a house such as lighting, heating, ventilation, and hot water. As a result, when these features are added to later models, it is expected that carbon and energy emissions will increase at first. This doesn't mean that the building is worsening in terms of energy performance, it simply builds a realistic picture of the energy and carbon of the building was to be used as a house, with varying scenarios in terms of heating and insulation for example. The different scenarios to be modelled using IESVE are shown in section 4.
4. Results & Discussion
4.1 Thermal imaging
The main sources of heat loss identified via infrared imaging are through the roof and windows, and since their collective area is so large, they can be said to be areas of particular concern. The windows are extremely old and are made of single panes of glass. Additionally, in most areas of the building, the glass is not securely bonded to the stone mullion surround. The roof is also a cause for concern, given that there is no insulation present, which is unheard of in buildings constructed today. Another main source of heat loss is via some coach doors, of which there are two sets, one set on the ground floor, and another set directly above these, on the first floor. These doors are large and are visibly in poor condition, which was confirmed using thermal imaging. The doors have been covered with carpet on the inside, to insulate them to some extent. Figure 3 shows the results of the thermal imaging assessment, detailing the areas of concern identified.
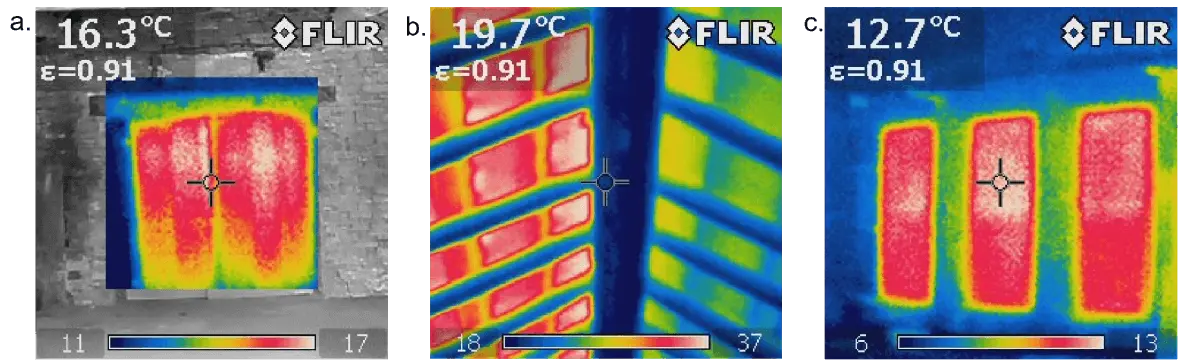
Figure 3. Thermal imaging results. (a) Heat loss via coach doors; (b) heat loss via roof; (c) heat loss via windows.
Figure 3a shows heat loss via coach doors situated on the first floor, however there is a second set of the same doors situated directly below, on the ground floor. The doors are made from timber which is in poor condition, and they have been covered in carpet on the inside, in a bid to offer a form of insulation from draughts. Figure 3a shows the poor fabric condition of the doors. These doors are 2.4 m wide by 2.2 m, hence this is a large area of heat loss. In addition to this, these doors are also a source of airflow due to their poor condition and the fact that they are poorly fitted, both of which cause a draught.
Figure 3b shows heat loss through the roof, this image confirms the lack of insulation in the building. The right-hand side of the roof is colder than the left side, indicated by the green colour for the cooler temperature and red to show the warmer temperature. The sun was incident on the left side of the roof, which accounts for the temperature difference between the left and right sides of the roof. Due to the phenomenon of heat rising coupled with the large area of the roof (when compared to the total area of the building) this is a large heat sink. Significantly, the highest temperature recorded in the whole building was through the roof, from the inside of the building.
Similarly, the windows in The Old Grammar School are allowing a lot of heat to escape through them. This is mainly due to the single glazing used in all the windows; the problem is exacerbated due to the poor condition of the glass and sandstone mullions. Figure 3c shows the heat loss through the glass, in addition to heat loss through the surrounding sandstone mullion, which is in poor visible condition as the sandstone can be seen to be crumbling.
4.2 Hygrothermal assessment
The schedule of data acquisition is shown in Table 2. In summary, data was recorded for a total of eight weeks. The data is organised into two sets, primarily due to the seasonal difference in temperatures due to the approach of summer. The data collected as part of set 1 was obtained when the weather was milder, whereas external temperatures were higher in June when the data was recorded for set 2. These two sets can then be compared to determine the effect of seasonal temperatures on the comfort level of the building.
Area Subject to Monitoring | Dates | |
Set 1 | Ground Floor | 23rd April-7th May |
First Floor | 7th May-21st May | |
Set 2 | Ground Floor | 2nd June-15th June |
First Floor | 15th June-29th June |
4.2.1 Hygrothermal differences between ground floor and first floor
The conditions on the ground floor can be compared with those on the first floor to demonstrate how heat and moisture move around the building. The following results are obtained by generating an average of all data across both sets for each floor. For example, the average temperature of the ground floor is obtained by calculating the average from the full 4-week data set for the ground floor, which was obtained during different months (i.e., set 1 and set 2). This was repeated for the other variables to compare data from the ground and first floors.
Table 3 shows the average temperatures for the ground floor and first floor over the period of eight weeks. The outdoor average temperature (April to June) of the studied building showed that the air temperature 15 ℃, which is relatively high compared to sensor readings as indicated in Table 4. However, outdoor relative humidity was 79% on average, which is slightly close to the one on the ground floor. This confirmed that the comfort level of the building needs to be improved.
Ground Floor | First Floor | |
Temperature | 11.96 | 14.85 |
Humidity | 77.45 | 71.15 |
Dew Point | 16.17 | 9.58 |
Model Number | Model Input |
1 | Baseline model with no lighting, auxiliary ventilation, with the only source of heating coming from open fires and no hot water in the building. Building layout modelled as open plan as it is in its current state, with no insulation present. |
2 | As model 1, but with the addition of partitions to create a layout in the form of a home. |
3 | As model 2, but with the addition of insulation to the building, in the form of polyurethane boards. |
4 | As model 3, but modelled as a home with auxiliary ventilation and hot water in the kitchen, bathroom, and downstairs toilet, as well as heating in the form of a gas boiler with under floor heating. |
5 | As model 4, but replacing the gas boiler with an air source heat pump and solar panels. |
6 | As model 5 but with the addition of double-glazed* windows*Using Pilkington Spacia or similar, having similar thermal properties to double glazed windows, but having a similar thickness to single glazing[13]. |
These results have been compared with the Figure S1 of CIBSE Guide A which is the recommended comfort level of specific applications[10]. As the current configuration of the building has no partitions and is just an open space segregated only by the floor divide between the ground and first floor, it has been decided that each floor will be compared with the recommended temperature for hall, stairs, and landings. This is because these areas are generally open spaces, which is the closest option that best represents the current space at The Old Grammar School. The recommended target temperature to achieve the comfort level according to CIBSE Guide A for halls, stairs, and landings is provided as a range of 19-24 ℃; the average value of this has been chosen for purpose of the comparison, which is 21.5 ℃. It is worth noting that the recommended temperature of halls, stairs and landings is lower than the recommended temperature for all other rooms in the home, such as bedrooms.
It is clear from this graph in Figure 4 that the temperature inside The Old Grammar School is significantly lower than the recommended temperature outlined in CIBSE Guide A. There is a particular concern with regards to the ground floor as temperatures here are much cooler than the first floor, yet the temperature on both floors falls short of the recommended temperature. These results are to be expected given that no insulation at all is present in the building, in addition to no heating system. Sandstone blocks are the main building fabric, and they are in very poor condition and can be seen to be visually crumbling. The mortar between blocks needs to be replaced and there are numerous inlets between the blocks. This means air and water can easily enter the building, hence keeping it cool. The first floor is of a higher temperature due to the phenomena of convection, and as a result warmer air rise. As the roof is not insulated, it absorbs heat during the day and hence the first floor is heated this way. Evidence of this is provided in the thermal imaging results, where the roof can be seen to be two different temperatures, with the side that is facing the sun having a higher temperature than the opposing side of the roof, which was shaded from the sun at the time of inspection.
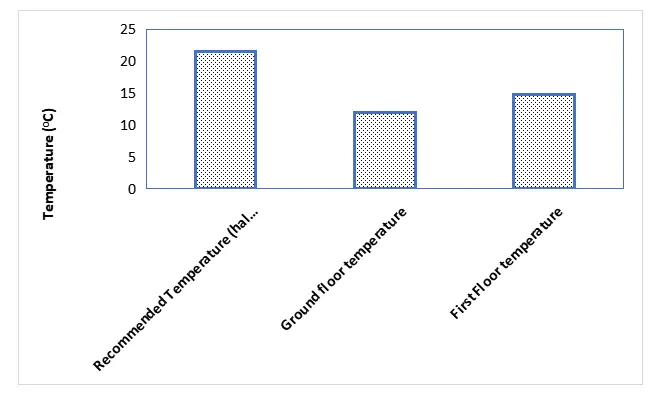
Figure 4. A graph of the comparison of recorded temperatures vs. those recommended in CIBSE Guide A. CIBSE: Chartered Institution of Building Services Engineers.
4.3 Environmental modelling
Environmental modelling was carried out to determine the current state of the building and create a baseline model. The main model outputs that are of interest are carbon emissions and total energy output. This model is then altered and the changes in carbon emissions and energy output are compared with one another. This method is used to find the best retrofitting solutions to improve emissions and energy use of the building. Table 4 details the different model scenarios that were created using the software.
4.3.1 Baseline model
A baseline model was created using the software IESVE to model the performance of the building in its current state during the whole year from January to February. This model was created to form a true picture of the current state of the building, in that no lights were added to the model, or auxiliary ventilation, and with the only source of heat being from four fireplaces that currently exist in the building. This was essential to create a baseline to improve upon. However, it is obvious that to convert the building into a house, equipment such as lights and ventilation would have to be added to the kitchen and bathrooms, as well as a heating system. Therefore, it can be expected that energy use will increase initially when fitting the building out with equipment required to make it a habitable space. Hence the results of the baseline model should be compared with other models with caution. Figure 5 shows 3D view of the baseline model of the building created with IESVE.
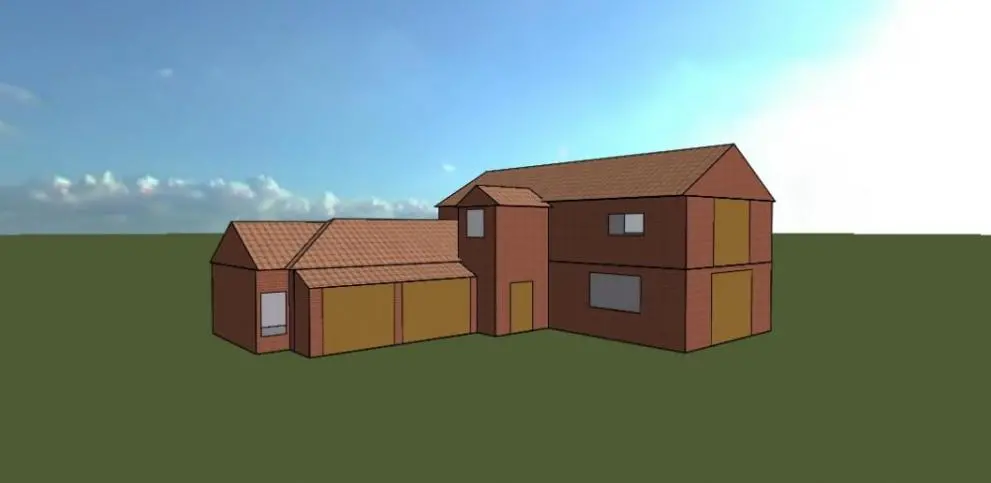
Figure 5. 3D view of the baseline model in IESVE. IESVE: Integrated Environmental Solutions Virtual Environment.
The options chosen for the creation of the baseline model are as highlighted under the experimental approach section. The use of ApacheSim (dynamic simulation) within IESVE generates a variety of model outputs. Outputs deemed significant for the baseline model include total system energy, carbon emissions, solar azimuth, and wind speed.
4.3.2 Total energy output
The highest value for total system energy is 10 MWh for the month of January and the lowest month is 0 MWh during the summer. This energy is comprised of heating, which is only in the form of open fires, there is no boiler, and no gas central heating. This energy also fails to consider auxiliary ventilation such as through extraction fans, which are usually found in bathrooms and kitchens, as there are no fans installed. Additionally, there is no lighting in the building in its current state. As a result, it can be said that this baseline model is not wholly realistic, because although the data that has been inputted into the model provides the best representation of the building as it currently stands, given the lack of lighting and inadequate heating in the building, it is not in a habitable condition. As a result of this, it is expected that the total system energy will increase when changes are made to the building to convert the building for residential use. It should also be noted that the template applied to the use of heating considers the building to be used as a domestic building, with a family, meaning that the property is vacant during the day, and hence heating and electricity will not be in use during normal working hours.
4.3.3 Total carbon emissions output
Another significant model output is the total carbon emissions. In this case, the carbon is produced entirely via the use of the heating, of which the only source is four open fires, which burn a smokeless fuel. Again, it is possible that the system carbon emissions may increase through the conversion of the building from industrial to residential use, given that lights and auxiliary ventilation need to be added. Similarly, to the total system energy, it is likely that the total system carbon emissions doesn't accurately represent the total carbon utilised, given that the building is currently not equipped with a satisfactory heating and ventilation system, which are required to make the building habitable. The model output shows that the total amount of carbon emitted through using fires for heating is equivalent to 22,312.73 kgCO2 per year. Again, the template applied is the same as the system energy, under the assumption that the building is occupied by a family that will not be inside the property during the average normal working hours throughout the week.
4.3.4 Weather outputs
Weather outputs are important in that they provide information that can be used when determining the feasibility of renewable energy sources that are dependent on the weather, which is the potential solar and wind power in this case. The desk-based site analysis using 'Geoindex' from the British Geological Survey did not identify the potential for any other sources of renewable energy at the site, such as geothermal.
Solar azimuth can be a useful model output. Solar azimuth is a measure of the angle of the sun. Solar azimuth is important as it can be used to determine the suitability of using photovoltaic energy in the form of solar panels in a specific location. The mean value of solar azimuth over a whole year is 182.3 degrees. This shows that the roof is South facing. Solar panels should have an orientation of 'west of east due south', and the optimum angle for a south-facing roof is between 20° and 30°[11]. Given that the roof of the building is south facing and that the angle of the roof is approximately 30°, it appears that solar energy is a feasible option as part of the retrofitting of the building.
Wind speed can identify the feasibility of utilising wind energy in a given area. The model outputs for windspeed show that the mean windspeed for the site of The Old Grammar School is 3.95 m/s, and the maximum windspeed to be expected at the site is 14.7 m/s, with a minimum windspeed of 0 m/s. An average wind speed of 7 m/s or greater is required to install a wind turbine[12]. As a result, wind speed at the site is not sufficient to take advantage of wind energy. Additionally, due to the potential for the turbines to be an eyesore, it is unlikely that planning permission would be granted due to the listing status and the location within a conservation area.
4.3.5 Proposed layout of home
The layout of the building was restricted as the partitioning required to create rooms could only be devised around existing windows and door frames, to keep the changes as minimal as possible due to the listing status. The layout chosen provides three bedrooms, a bathroom and downstairs toilet, as well as two living rooms and a kitchen. A plan of the ground floor is shown in Figure 6, whereas the first-floor plan is shown in Figure 7.
4.3.6 Modelling results
The baseline model is referred to as model 1. Changes were then made to this model to create different model scenarios to see which type of changes had the most significant impact on the carbon emissions and energy output of the building. The overall aim of this was to reduce carbon emissions and energy output as much as feasibly possible. The models can be compared to one another, as well as the baseline model and more importantly with the industry benchmarks. The models are numbered from 1-6 and the different model inputs are detailed in Table 4 below.
4.3.7 Energy and carbon outputs
The results show the energy consumption for gas and electricity in KWh and corresponding carbon emissions in kgCO2 from the modelling of the six different scenarios, which are given in Table 5 below. Each scenario showed the impact of applying a certain solution to improve energy performance and comfort level. This was reflected by reducing the amount of energy and carbon in the different scenarios compared to the baseline.
Model | Total Energy Output (kWh) | Total Carbon Emissions Output (kgCO2 e) | ||
Max | Mean | Max | Mean | |
(1) Baseline Model | 46.6 | 5.88 | 20.2 | 2.5 |
(2) Baseline Model + Partitions (without insulation) | 52.7 | 6.43 | 22.8 | 2.8 |
(3) Model with partitions with insulation | 42.5 | 4.60 | 18.4 | 2.0 |
(4) Model with partitions, insulation, lighting, auxiliary ventilation and gas boiler, hot water | 50.04 | 9.54 | 10.8 | 2.1 |
(5) Same model but with renewable energy (air source heat pump & solar panel) | 19.38 | 3.34 | 10.1 | 1.7 |
(6) Same as above but with double glazed*windows, insulation at roof and internal ceiling/floor*Using Pilkington Spacia or similar, having similar thermal properties to double glazed windows, but having a similar thickness to single glazing[13] | 18.33 | 3.45 | 9.5 | 1.8 |
The energy and carbon emissions increase when partitions are added on top of the baseline model (Model 2). This is due to the impact of the partitions on the hygrothermal behaviour of the building. Energy is required to heat the different rooms in the building, when compared to heating one open space.
For model 3, when insulation was added, the energy and carbon emissions decrease as heat is retained in the building, as well as preventing the ingress of cold air. This creates more comfortable conditions within the building, with fewer fluctuations in temperature.
Model 4 is the first model that was created with all the equipment needed to make the space habitable and with the potential to be used as a home. As a result of this, energy and carbon emissions increase when compared to the previous models. This is due to the addition of lighting, auxiliary ventilation, hot water, and a proper heating system in the form a gas boiler. All this equipment requires energy to run and hence the energy and carbon emissions have increased for this model, when compared to model 3.
Model 5 is the same as model 4, however the gas boiler from model 4 is replaced with solar panels and an air source heat pump. The replacement of the gas boiler with renewable energy reduces the energy output significantly, and energy output more than halves when using renewables. The average carbon emissions are also less in model 5, albeit the reduction is not as great as the reduction in energy emissions.
To improve upon model 5, insulation was added in the form of polyurethane boards to the internal ceiling/floor, as well as at the underside of the roof. Additionally, the windows were improved too - as the building is listed, double glazed windows cannot be added. However, Pilkington Glass produce a type of window called Pilkington Spacia which performs similarly to double glazing, yet it is as thin as single glazing[13]. As this type of glass would improve thermal performance at the cost of minimal cosmetic changes to the building, this was chosen to add to model 6. The results from model 6 show a very slight improvement with regards to both carbon emissions and energy output. As a result of the small improvement, it is likely that any further retrofitting will not have a large impact on carbon emissions and energy output. This is because a certain amount of energy is always going to be required to keep the building operational and habitable, for equipment such as lighting and heating. The final results for model 6 show the energy output has more than halved compared to model 1, whereas the carbon output of model 6 is half of the output in model 1. This proves the retrofitting measures to be successful in reducing the carbon emissions and energy output, whilst making the building habitable at the same time by installing the necessary equipment needed to convert the building into a home.
The energy output and carbon emissions both show an increase in model 2, this model is the same as the baseline i.e., there is still a lack of a proper heating system, lighting, and hot water, however this model includes partitions, instead of the open plan which can be seen in model one. Hence this clearly shows that adding partitions greatly impacts the carbon and energy emissions of the building, perhaps due to how the partitions alter the hygrothermal conditions. Heat cannot dissipate freely throughout the building when the area is segregated into rooms, and this clearly has a huge impact on the carbon and energy emissions which is due to the extra heating that is required to heat the building when it is partitioned.
In terms of the carbon, model four shows the first significant decrease in emissions, which may appear somewhat surprising at first, as this model is the first model to have lighting, ventilation, hot water, and heating in the form of a gas boiler and radiators. Clearly one would expect emissions to increase when adding this equipment to the building, however, as the building is equipped with a gas boiler and radiators in this model, the different rooms within the building appear to maintain heat for longer, this explains the trend with regards to the carbon emissions.
Models 4-6 show minimal decreases with regards to carbon emissions, and as a result the results are plateauing and hence it is unlikely that carbon emissions can be significantly reduced any further than this. Overall, when looking at the maximum carbon emissions, the emissions have more than halved when comparing the baseline model to the final design, which is model number six. This shows there is potential for a great improvement with regards to carbon emissions from the building by making the necessary retrofitting improvements to the building. These improvements are the addition of lighting, auxiliary ventilation in the kitchen, toilets, and bathrooms, as well as solar panels and the use of an air source heat pump.
4.4 Comparison with benchmarks
The results thus far show an improvement in energy output and carbon emissions of the building through the simulation of different retrofitting options. To determine if these improvements are enough to consider the building to be an eco-home, the results must be compared against industry benchmarks set out by CIBSE.
Model 5 is compared against industry standard benchmarks to assess the performance of the proposed eco-home conversion. The baseline model cannot be compared against benchmarks due to the insufficient services inside the building, such as lack of a proper heating system, and hot water, hence making the building inhabitable. Nonetheless, it is important to compare the proposed eco-home design against benchmarks to assess the performance of the retrofitted building. Table 6 shows the Government Greenhouse Gas Conversion Factors for 2021. Greenhouse gas conversion factors are used to calculate emissions for certain activities, and new factors are produced annually[14].
Category | KgCO2 |
Electricity | 0.21233 |
Gas | 0.24677 |
These factors are then multiplied by the actual emissions of the building, or in this case the model outputs for emissions as generated by IESVE. These results can then be used in conjunction with the benchmarks set out by CIBSE in CIBSE TM46.
Table 7 shows the Energy and CO2 benchmarks as set out in CIBSE TM46, which sets out energy benchmarks for different categories of buildings. It is important to note that CIBSE TM46 is recommended for non-domestic buildings. However, given the nature of the Old Grammar School being used as an industrial building for hundreds of years, and that its dimensions are not typical of those of a home, it is still beneficial to use CIBSE TM46. Considering this, the benchmark category chosen from TM46 is for long term residential, as this was the category that was the closest representation of the proposed eco-home. In addition to this benchmark, the DECC in the UK has set heat benchmarks for homes, so the proposed eco home design from model 6 can also be compared with the benchmark set by DECC.
Energy Benchmarks | CO2 Benchmarks | ||
Electricity typical benchmark(kWh/m2) | Fossil-thermal typical benchmark(kWh/m2) | Illustrative electricity typical benchmark (kgCO2/m2) | Illustrative fossil-thermal typical benchmark(kgCO2/m2) |
65 | 420 | 35.8 | 79.8 |
CIBSE: Chartered Institution of Building Services Engineers.
Model 5 is significant since this model shows the building equipped for habitation in that this model has hot water, auxiliary ventilation, lighting, and heating, however this model is the first model to take advantage of renewable energy. Model 5 uses solar panels, which occupy an area of 27 m2 on the south facing elevation of the roof. This is approximately 75% of this face of the roof, which is equivalent to around 38% of the entire roof space, providing enough space around the edge of the roof for access. The results of this model are compared to the benchmarks and are shown in Table 8 below.
Electricity (kWh/m2) | Carbon (kgCO2/m2) | ||
Benchmark | Actual | Benchmark | Actual |
65 | 169.8 | 35.8 | 36.06 |
CIBSE: Chartered Institution of Building Services Engineers.
These results show that for carbon emissions, the proposed design detailed in model 5, produces results only slightly above the baseline. Whereas for electricity, the baseline value is far exceeded. This is expected, as there is no gas usage at all, which generally means the lack of gas is compensated for by an increase in electricity, as all heating is provided by electricity. Secondly, electricity use increases due to the methods of energy production used in the building, using solar panels and an air source heat pump. These require electricity to run, and the energy produced by these renewables is utilised in the form of electricity. As a result, using renewable energy does lead to an increase in electricity use, although the electricity is from a sustainable source, and hence the results being above the baseline are not as alarming as they may appear at first.
Moving forward, to further improve upon these results, the building could be maximised in terms of insulation. In prior models, insulation is installed in the form of polyurethane boards on the inside of external walls. This can be optimised by also installing insulation on the internal floor and ceiling, as well as at rafter level. Additionally, although double glazing is not permitted due to the listing status of the building, there are windows available on the market that are of a similar thickness to single glazing, yet they perform as well as double glazing, such as the Pilkington Spacia glass[13]. These changes were made as part of model 6, and then these results were then compared to the baseline again. Table 9 shows the model 6 outputs compared with the baseline.
Electricity (kWh/m2) | Carbon (kgCO2/m2) | ||
Benchmark | Actual | Benchmark | Actual |
65 | 138.8 | 35.8 | 29.4 |
Table 9 shows that carbon emissions are now better than the benchmark, also strictly speaking gas use is better than the benchmark, as there is no gas used in the building. Electricity usage is above the benchmark but as previously discussed, this is expected due to the use of renewable energy to heat the house, and to compensate for the lack of gas usage. As the final proposed design performs better than the benchmark on two out of the three criteria, that is carbon emissions, electricity usage and gas, then the proposed design is considered to be eco-friendly. Figure 8 shows the electricity usage from the final design (model 6) compared to the benchmark.
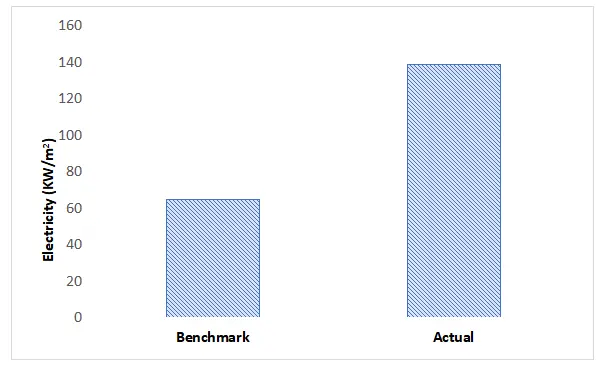
Figure 8. A bar chart comparing the actual electricity usage from model 6 compared to the benchmark.
Electricity is above the benchmark as already outlined, yet this should not be a cause for concern. Figure 9 shows the carbon emissions projected by model 6, compared to the benchmark. As shown carbon emissions are almost 1/5 less than the benchmark, showing great savings in carbon emissions.
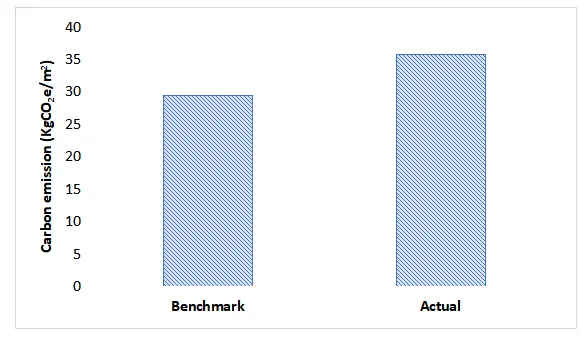
Figure 9. A bar chart showing the actual carbon emissions from model 6 compared to the benchmark.
The results for model 6 can now be compared to the benchmarks set out by DECC. Table 10 shows DECC baselines for a pre 1917 domestic property, compared to the electricity usage for model 6.
Actual electricity usage (kWh) | Semi-Detached baseline (kWh) | Detached baseline (kWh) |
26,770 | 20,476 | 30,714 |
DECC: Department of Energy and Climate Change.
The actual electricity usage has been compared to the baseline for a detached and semi-detached home. This is because The Old Grammar School was constructed as a detached building, however when Old Grammar School Terrace was built in the late 1800s, a house was attached to one side of the building. It is decided that the property will be compared to the detached property baseline, as the building was constructed as a detached property and hence doesn't have thinner internal walls where the property meets an adjacent house, which would usually be the case for a semi-detached property. The electricity use is less than the baseline for a detached property, but slightly above the baseline for a semi-detached property. It should also be pointed out that the baselines are different for homes constructed in different decades, with the oldest baseline classified as pre-1917, which is over 200 years after The Old Grammar School was constructed, hence it is possible that these baselines were not designed to be used for homes that are as old as The Old Grammar School. Nonetheless, it is beneficial to compare the results as it is likely that the baselines are more stringent, when using them for a property that is over almost 350 years old.
5. Conclusion
This study has met the research outcomes for several reasons. Firstly, the current performance of the building has been established, the hygrothermal assessment showed that the humidity of the building is outside of the normal range for a typical building, however it is just bordering the acceptable limit for historic buildings. This is something that should be assessed again after repairs have been carried out to make the building watertight. The hygrothermal assessment also revealed that the temperature of the building is below the comfortable range when compared with the recommendations set out in CIBSE Guide A, this a significant indicator of the comfort within the building, suggesting improvements need to be made if the building is to be used as a home. Thermal imaging revealed that the main sources of heat loss in the building were from the roof, which is uninsulated, as well as through the windows which are single glazed. As a result, these areas should be targeted when implementing retrofitting. The results from these initial assessments impacted the different model scenarios chosen. In terms of the modelling, after the creation of the baseline model, partitions were added to convert the open space into rooms typical of a house. The partitioning proved to have a significant impact on the energy output and carbon emissions and adding these to the baseline caused energy and carbon emissions to increase significantly. The 'eco-energy efficient home' design consisted of all the equipment required to convert the building into a home. When compared to the baseline, this design proved to have fewer emissions than the baseline model, even with the addition of all the equipment to make the building habitable, which was not inputted into the baseline model. The model outputs of the final design were compared to different industry benchmarks including those outlined in CIBSE TM46, as well as those provided by DECC. The final design showed emissions to be lower than the benchmark for carbon emissions and gas, whereas electricity emissions were higher than the benchmark; perhaps due to the use of electricity used for heating due to the renewable energy used. The design also performed better than the benchmark as set out by DECC. Given that out of the four benchmarks, the design performed better than the benchmarks (i.e., emissions are lower than the benchmark) on three out of the four criteria, it can be said that it is possible to convert The Old Grammar School into an eco-home, although it is recognised that planning permission must be granted for this change of use and to allow the retrofitting options outlined in the final design.
Authors contribution
All authors contributed equally to this work.
Conflicts of interest
The authors declare no conflicts of interest.
Ethical approval
Not applicable.
Consent to participate
Not applicable.
Consent to publication
Not applicable.
Availability of data and materials
Not applicable.
Funding
None.
Copyright
© The Author(s) 2024.
References
-
1. Mulheirn I [ Internet]. Tackling the UK housing crisis: is supply the answer. UK Collaborative Centre for Housing Evidence; 2019 [cited 2021 Jul 19]. Available from: https://www.institute.global/insights
-
2. HOME BUILDERS FEDERATION [Internet]. New build homes save owners £629 a year on energy bills; 2017 [cited 2021 Jul 19 ]. Available from: https://www.hbf.co.uk/news/new-build-homes-save-owners-629-a-year-on-energy-bills/
-
3. NEW-HOMES WEEK [Internet]. Customer Protection; 2020 [cited 2021 Jul 19]. Available from: https://www.new-homes.co.uk/why-buy-new/customer-protection/
-
4. Grant A, Ries R. Impact of building service life models on life cycle assessment. Build Res Inf. 2013;41(2):168-186.
[DOI] -
5. Kollewe J. More than half of new-build homes in England 'have major faults' plus the best city cycle schemes [Internet]. London: The Guardian; 2017. Available from: https://www.theguardian.com/
-
6. Berg F, Fuglseth M. Life cycle assessment and historic buildings: energy-efficiency refurbishment versus new construction in Norway. J Archit Conserv. 2018;24(2):152-167.
[DOI] -
7. Gong Y, Song D. Life cycle building carbon emissions assessment and driving factors decomposition analysis based on LMDI - A case study of Wuhan City in China. Sustainability. 2015;7(12):16670-16686.
[DOI] -
8. Zhour F, Abdellatif M, Osman Y, Lee D. Comparison between the life cycle carbon footprint of refurbished and new buildings: a case study of Community Centre. J Build Des Environ. 2023;2(1):13597.
[DOI] -
9. HISTORIC ENGLAND [Internet]. Old Grammar School, to rear of numbers 8-14 (numbers 8-14 not included); 2021 [cited 2021 Mar 18]. Available from: https://historicengland.org.uk/listing/the-list/list-entry/1220218
-
10. Chartered Institution of Building Services Engineers. Environmental Design CIBSE Guide A. London: Chartered Institution of Building Services Engineers; 2021.
-
11. Hailu G, Fung AS. Optimum tilt angle and orientation of photovoltaic thermal system for application in greater Toronto area, Canada. Sustainability. 2019;11(22):6443.
[DOI] -
12. RENEWABLES FIRST [Internet]. How windy does it have to be? 2015 [cited 2021 Aug 25]. Available from: https://renewablesfirst.co.uk/renewable-energy-technologies/windpower/windpower-learning-centre/wind-turbine-fundamentals/#top
-
13. PILKINGTON [Internet]. Listed building transformed with new energy efficient Pilkington Spacia glazing; 2019 [cited 2021 Aug 25]. Available from: https://www.pilkington.com/en-gb/uk/news-insights/featured-articles/listed-building-transformed-with-new-energy-efficient-pilkington-spacia-glazing
-
14. UK GOVERNMENT [Internet]. Government conversion factors for company reporting of greenhouse gas emissions; 2021 [cited 2021 Aug 25]. Available from: https://www.gov.uk/government/collections
-
15. Department of Energy & Climate Change [Internet]. Assessing the cost effectiveness of individual metering: Energy demand benchmarks; 2014 [cited 2021 Aug 19]. Available from: https://assets.publishing.service.gov
-
16. Chartered Institution of Building Services Engineers. TM46 Energy benchmarks (2008). London: Chartered Institution of Building Services Engineers; 2008.
Copyright
© The Author(s) 2024. This is an Open Access article licensed under a Creative Commons Attribution 4.0 International License (https://creativecommons.org/licenses/by/4.0/), which permits unrestricted use, sharing, adaptation, distribution and reproduction in any medium or format, for any purpose, even commercially, as long as you give appropriate credit to the original author(s) and the source, provide a link to the Creative Commons license, and indicate if changes were made.
Share And Cite