Abstract
Utilizing recycled aggregate concrete (RAC) is important because it solves the problems of resource scarcity and pollution from urban waste. Using RAC will have a significant positive environmental impact over time. Many studies compare the environmental benefits of RAC with natural aggregate concrete (NAC) and use life cycle assessment (LCA) to analyze the benefits of RAC. However, a mature and comprehensive research system for LCA application in RAC has yet to be developed. The purpose of this study is to review the environmental impacts of RAC throughout its life cycle using the novel perspective of the four steps of LCA, identify methods to address or mitigate biases, and suggest future development directions for this technology and database improvement to provide useful references for future research. The findings show that all four stages of LCA (goal and scope definition, life cycle inventory analysis, life cycle impact assessment, and life cycle interpretation) have issues, primarily related to data measurement and selection, process step assumptions and simplifications, and algorithm limitations. We also recommend using the life cycle basic function evolution method to optimize Monte Carlo simulations, which reduces the uncertainty of LCA results. In the future outcomes of LCA ought to emphasize on the carbonation process and the analysis phase of cement's second life cycle.
Keywords
1. Introduction
Concrete is a popular artificial material around the world, playing an important role in a variety of fields due to its versatility, accessibility, and inexpensiveness. However, the environmental consequences of large-scale production and widespread use of concrete have become increasingly apparent, particularly since cement production has become a significant contributor to global warming[1]. According to statistics, concrete waste accounts for approximately 34% of all Construction and Demolition (C&D) waste generated each year worldwide[2]. In 2022, global C&D waste exceeded 3 billion tons, with concrete waste being the most common[3]. The United States generates 85% of C&D waste, Australia 81%, and China 45%[3]. China generates approximately 2.4 billion tons of C&D waste each year, which accounts for 40% of total urban solid waste[4]. Concrete waste accounts for the majority of C&D waste (60-70%), and is expected to reach 7.75 billion tons globally by 2050[5]. It is worth noting that traditional concrete manufacturing, particularly Portland cement production, contributes significantly to environmental degradation. The cement industry alone generates 5-8% of global CO2 emissions, which are directly related to climate change[6]. Given the growing scarcity of global resources and severe environmental pollution, effectively treating and utilizing waste concrete to achieve resource recycling has become an urgent priority. Under these conditions, research into Recycled Aggregate Concrete (RAC) has emerged[2].
Energy conservation and environmental protection are critical to ensuring long-term economic development[7,8]. Green buildings, which aim to maximize resource savings, protect the environment, and reduce pollution throughout the building's life cycle, have become an unavoidable trend in the construction industry[9]. Recycled Concrete Aggregates (RCAs) (Figure 1) are a sustainable civil engineering material made primarily from the demolition of buildings and infrastructure, which includes crushed concrete, bricks, and other waste. The construction industry accounts for 50% of all extracted materials globally, with sand and gravel being the most common, and sand is the second most consumed resource after water[11]. By converting these into usable resources, RCAs are expected to alleviate raw material shortages while also improving environmental performance in concrete production[12]. A research has assessed how well different fly ash addition rates work in place of cement in RAC[13]. The test results at different ages (7, 28, and 90 days) indicate a significant improvement in concrete's performance when 20% fly ash is used[13].
In result, there is an urgent need to comprehensively evaluate the environmental impacts of RCAs, and life cycle assessment (LCA) is an appropriate method for quantifying the direct and indirect environmental loads of their utilization process. LCA is a systematic approach that evaluates a product or system's potential environmental impacts over its entire life cycle. LCA is a standardized method for quantifying the potential environmental impacts of materials, services, products, and processes. In 2006, the International Organization for Standardization published standards for LCA implementation, known as ISO 14040 and ISO 14044. ISO 14040 establishes the principles and framework for the management of LCA, whereas ISO 14044 specifies the requirements and guidelines. The primary contribution of these standards is to define LCA's four stages: goal and scope definition, life cycle inventory (LCI) analysis, life cycle impact assessment (LCIA), and interpretation[14]. LCA may cover all stages of RAC[15]. LCA converts the environmental load (e.g., pollution emissions and resource consumption) generated by RCAs throughout their life cycle into comparable scores using characterization factors, allowing us to better understand the potential environmental impacts of upstream and downstream activities[15]. It can assess the potential impact of various environmental issues (such as ecological balance, human health, environmental safety, resource or energy consumption)[16]. Depending on the goal and scope of the LCA study, some stages may be prioritized over others[17]. Although some scholars have used LCA to assess the environmental impacts of RAC[18], there are still numerous challenges to practical application. Specifically, the evaluation process of LCA is complex, and the results of LCA are easily influenced by various parameters that must be carefully considered. These parameters include the selection of functional units, the definition of system boundaries, and the quality control of input data[3]. Furthermore, subjective factors limit LCA application, such as misunderstandings and limitations of the life cycle approach, as well as a lack of incentives and motivation to use such methods. These factors restrict the widespread application of LCA to some degree. As a result, while adopting LCA for RAC environmental evaluation is important, we must face numerous challenges[3]. As a result, the purpose of this paper is to thoroughly examine the issues raised in the environmental impact assessment of RAC from four perspectives (goal and scope definition, LCI analysis, LCIA, and interpretation), and to make recommendations for how to address them.
2. Literature Review
For the review, this work employs the research methodology suggested by Vega et al.[17]. To ensure comprehensiveness and accuracy, we thoroughly researched relevant literature and papers, combining Chinese resources such as the China National Knowledge Infrastructure database to cover a broader academic field. During the literature selection process, we concentrated on key information such as topics, authors, and keywords to ensure that the chosen literature was highly relevant to our study. The five search keywords used were "life cycle assessment or LCA", "recycled aggregate concrete or RAC", "recycled concrete aggregate or RCA", "environmental impact or environmental performance", and "sustainability," as well as their combinations, to search and identify the reviewed literature. Using the LCA details and the direction of use for RCAs as evaluation criteria, we performed a full-text evaluation of the retrieved literature in this study. The final selection consisted of approximately 40 representative papers for in-depth analysis. Journal papers were the most common type of publication in the selected literature body, followed by book chapters, doctoral and master's theses. In order to methodically investigate the problems raised by the LCA of RAC, we further grouped and classified the literature based on the stages of the LCA.
According to ISO 14040 and ISO 14044, LCA is divided into four stages: goal and scope definition, LCI analysis, LCIA, and interpretation. This study examines these four phases from a fresh angle not found in previous research, emphasizing the disagreements and problems with the LCA of RCAs. We point out future directions for technological development and offer solutions. Furthermore, this review can be used as a guide by future investigators studying the LCA of RAC.
3. Goal and Scope Definition
The first step in performing the LCA of RAC is defining the goal and scope, which also acts as the basis for further analyses. One crucial component of this process is selecting the functional unit. The product's function is described both qualitatively and quantitatively by the functional unit, which also has a major impact on the environmental impact assessment's findings[19]. Furthermore, during the evaluation, a variety of the product's performance aspects ought to be taken into account. Concrete volume was primarily used as the functional unit in early studies of LCA[20,21]. This definition makes data management and application easier, but because it ignores the strength and durability of concrete, it introduces errors into impact assessment results. This paper argues that the definition of the functional unit should take concrete performance attributes like strength, durability, and strength reliability into account in addition to volume, based on recent research[22]. Furthermore, as the most important and direct indicator of concrete performance, compressive strength ought to be a crucial component of the functional unit[23]. Notably, the percentage of concrete in the material inventory will decrease when the functional unit is a bridge or a sizable, finished building. This could result in errors in the overall impact assessment of RCAs[1]. The functional unit is a quantitative unit in the LCI process, so the functional unit definition must satisfy both the research requirements for cement properties and accurately reflect environmental impacts.
LCA can be broadly classified into two categories based on the scope of the research: "cradle-to-grave" and "cradle-to-gate". The former speaks of the overall emissions of pollutants into the environment from the extraction of raw materials through the production, distribution, use, and eventual disposal of products. The term "Cradle-to-gate" refers mainly to the total greenhouse gas emissions from the extraction of raw materials through the manufacturing of products and the output of factories. The research we reviewed indicates that "cradle to gate"[24,25] is the mainstream system boundary setting used in studies on RCAs. This boundary covers the processes of extracting and transporting raw materials, producing cement, and using related energy[26] (Figure 2). Furthermore, a number of recent studies have widely accepted the idea of "cradle-to-cradle" system boundaries[27].
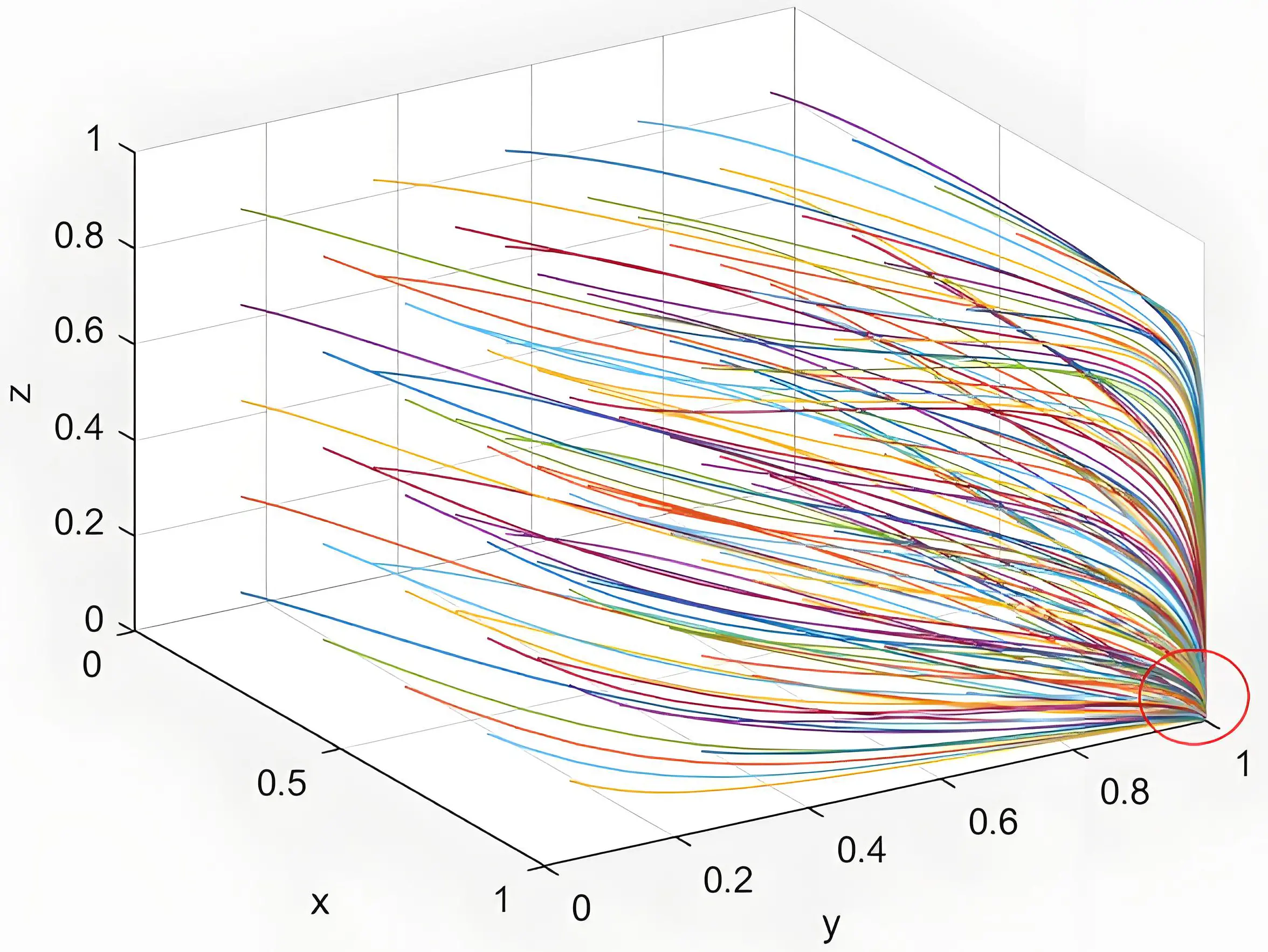
Figure 2. System boundary inventory for RAC. Revised from[26]. RAC: recycled aggregate concrete.
Notably, the database we have selected presents a new boundary known as "cradle-to-laying"[28], where "laying" refers to the process of building pavement with RCAs. This novel boundary is also classified as "cradle-to-gate" based on our comprehension of system boundaries and comparison with other literature. It is noteworthy that disparities in impact assessment outcomes may arise from the omission of specific steps in the production and utilization of RAC due to varying system boundary settings.
The 2018 China Building Materials Federation publication "Methods and requirements of low-carbon products evaluation for ready-mixed concrete" serves as an example of the low-carbon footprint concrete evaluation process in the "cement-concrete" product system. The carbon emission limits for concrete products are outlined in this standard, which also suggests fundamental guidelines for determining life cycle carbon footprints[29]. This method has the advantage of having a relatively well-developed evaluation approach, which aids in the formation of standardized guidelines for broad use. Furthermore, this method provides an intuitive approach to assessing the carbon emissions of concrete products. This approach does have certain drawbacks, though. First, carbon footprint calculations require high standards from databases and LCA models, which means specific systems must be established for controlling databases and the calculation process. Second, while the carbon footprint can accurately measure the carbon emissions of concrete products, it is difficult to fully reflect the carbon reduction effects of some high-performance concrete products across the entire building life cycle using product carbon footprints alone. This means that the approach has difficulties when it comes to handling comprehensive system assessments. This approach does, however, have certain drawbacks. First of all, complex systems must be established to constrain the databases and calculation process because carbon footprint calculations require high quality LCA models and databases. Second, while measuring the carbon footprint of concrete products is useful, it can be challenging to fully quantify the impact of high-performance concrete products' ability to reduce carbon emissions over the course of a building's life cycle. This suggests that addressing comprehensive system evaluations presents difficulties for the approach.
Waste heat recovery technology has been widely used in the energy-saving and emission-reduction modifications that some businesses have made to cement production processes in recent years. Specifically, about half of the electricity used in cement production comes from waste heat recovery power generation systems. Some studies may overlook or oversimplify the raw material extraction and transportation stages due to their relatively low environmental load and the challenge of obtaining relevant data.
4. LCI Analysis
The LCI stage of LCA involves compiling and quantifying the inputs and outputs for the product under study over its whole life cycle. Data is collected and analyzed at this stage for every stage of the product's life cycle, including the extraction of raw materials, production, transportation, use, recycling, and disposal. We are able to comprehend the energy consumption, resource consumption, and pollutant emissions at every phase of the product's life cycle by examining these data[30]. The idea of inventory analysis has been modified to fit particular situations. According to Xie[31], LCI analysis is the step in LCA where inputs and outputs are gathered and quantified for the product under study over its whole life cycle. LCI is the process of objectively quantifying resource inputs and environmental outputs, according to Zhu et al.[32].
The following actions are necessary for the inventory analysis:
1) Data Collection and Calculation: The inventory analysis process begins with this. It mostly entails gathering all relevant input and output data for the product being studied and carrying out computations. Li et al.[26] chose a product from a Shanghai-based RCA manufacturing facility, examined the production process, established system boundaries, and calculated the mix proportion of RAC based on a literature review (Table 1).
Materials | Proportion (kg·m -3) |
Cement | 485 |
Silica fume | 49 |
Limestone powder | 186 |
RCAs | 884 |
River sand | 755 |
Water | 128 |
Water reducing agent | 3.15 |
RAC: recycled aggregate concrete.
2) Classification and Quantification: Classification is the process of arranging inputs and outputs in a way that makes them easier to analyze and evaluate later on. The process of translating grouped inputs and outputs into precise numbers or ratios is known as quantification.
Normalization: In this step, each input and output's quantified values are converted into units that are comparable. For example, converting all quantified values to the same unit, such as kilograms, allows for comparison across different indicators.
Assessment: In this step, the quantified inputs and outputs are used to evaluate the environmental impact. For example, estimating their effect on acid rain and global warming potential. It should be noted that the steps listed above are flexible and can be altered to suit specific requirements. For instance, filtering and processing data based on particular characteristics and research directions is required during the data collection and calculation phase. Depending on the product's life cycle, various input and output classifications and quantification may be needed during the quantification phase.
Databases are the primary source of data used in LCA of RCAs. Local suppliers and manufacturers provide the missing data. Of these, Ecoinvent is the most used database, involving roughly 70% of the chosen literature[12,14,17,22,23,29,33-39]. Certain studies employ primary analysis data from local manufacturers and suppliers[1,2,7,19], while other studies use databases from other sources[40,41], such as Gabi. Inventory content includes not only databases, but also material allocation. Some researchers concentrate on the allocation of output products in order to better quantify and distinguish the resource consumption and environmental impacts of primary and byproducts. The methods for allocating RCAs in the inventory are primarily classified as mass allocation[40] and market value allocation[19,40]. Furthermore, the content of the inventory is related to the cut-off rules that the researchers have chosen. Vega et al.[28] handled RCAs using the "cut-off" allocation method, omitting the transportation and removal of pavement materials. Using the "treatment of waste concrete, not reinforced, sorting plant | waste concrete, not reinforced | cut-off" waste flow, Cao et al.[34] chose the data for waste concrete disposal in the database. After defining the functional unit, the consumption of NCAs typically needs to be higher than that of RCAs in order to ensure the physical properties of RAC (such as hardness and rut resistance), regardless of how inventory data content changes[1,12,28,29,42]. Section six explains the causes of this phenomenon. Notably, in order to compare variations in physical properties or environmental benefits, some studies have set up experimental groups where the consumption ratio of NCAs is higher than that of RCAs[27,36,43].
Despite numerous related studies, there is still much room for improvement and refinement in the LCI analysis process. Determining the transportation distance is especially important. Transportation distance significantly affects the environmental impact assessment's results in the LCA of RAC. According to Zhang et al.[19], it's important to consider the mode of aggregate transportation (road, rail, or waterway), the type of concrete, and the distance between the production and construction sites. Aggregate transportation relies on roads, railways, or waterways, with varying environmental impacts[19]. According to Pu et al.[23], carbonated RAC has a significant environmental impact due to cement production and transportation. As a result, researchers have consistently focused on transportation distance in the LCA of RAC. To ascertain the environmental impact of varying transportation distances for RAC and NAC, Kleijer et al.[44] performed a comparative analysis of "restricted" transportation distances. An deep comparative study of stationary and mobile plants processing concrete waste to create RAC was carried out by Mostert et al.[40]. Although transportation distance has always been a focus in environmental impact assessments, accurate determination and calculation in practice frequently falls short of ideal requirements. One major reason is regional differences, as cement production sites and raw material sources differ. Transportation distances differ significantly between regions[40]. In areas with sufficient supply of NCAs and nearby concrete production facilities, the transportation distance of aggregates and concrete may be relatively short[40]. Since all concrete is produced in one location, Jiménez et al.[1] demonstrated that changes in transportation data are primarily dependent on the amount of material transported rather than the distance from suppliers. A different study found that the longer transportation distance of NCAs is the primary reason why RAC has a lower environmental impact than regular concrete blocks[45]. As a result, the research findings regarding transportation distances in various regions differ significantly. While LCA typically demands practical inventory data to produce more accurate evaluation results, going overboard with practicality can seriously compromise the analysis's representativeness and applicability. To guarantee the validity and accuracy of the assessment, a balance between the practicality of the data and the generalizability of the findings must be found during the evaluation process. An environmental impact assessment of a particular fixed transportation scenario was carried out by Pradhan et al.[33], however this method limited the study's scope and might not have allowed for a thorough adaptation and assessment of the environmental impact under alternative scenarios[33]. When conducting an LCA for RCAs, this is one of the typical problems[33]. As of right now, no practical answers have been discovered. In the future, we hope to find more effective ways to tackle this problem.
Furthermore, we observe that the pursuit of data accuracy still has certain gaps. Certain studies consider the transportation distance to be zero since they assume that the water source is obtained directly from the site[42]. Other studies suggest that upstream transportation of construction material waste to the recycling site does not need to be considered[35]. Additionally, some studies make the assumption that every component is delivered by truck[35]. Vega-Araujo et al.[28] handled RCAs using the "cut-off" allocation method, which means that the inventory analysis did not account for the environmental effects of pavement removal and the transportation of recycled materials. The lack of adequate theoretical foundation for the assumptions made in certain literature to streamline the computation of transportation distances significantly undermines the validity of the evaluation findings. To address the problem, we drew on a variety of calculation methods from the literature and made more reasonable recommendations. When determining transportation distance inventory data, we recommend including as many transportation tools, fuel types, and transportation times as possible. Statistical probabilities should be used to weight various transportation schemes in order to generate more persuasive representative data. This allows the specific impact of transportation distances to be better reflected in subsequent impact analyses, improving the accuracy and reliability of the evaluation results. The mix ratio of RAC to NC must then be taken into account. Previous research indicates that an appropriate reduction in the water-binder ratio is required when formulating the mix ratio of RAC because of its relatively weak performance[46,47]. This indicates that a suitable increase in cement usage is necessary to maintain a compressive strength comparable to freshly mixed concrete[46,47]. It is important to keep in mind, though, that the percentage of NAC in RAC will vary depending on the requirements of various regions regarding cement mix ratios and particular cement uses. According to Ding et al.[27], the cement proportion has continuously contributed the most to all NAC and RAC impact categories. Thus, the first thing that needs to be addressed is figuring out the proper cement ratio. The Marshall mix design method has been employed in certain studies to assess the environmental impact of varying proportions of RCA mixtures[38]. Following this, additional research has looked into mechanical characteristics like rutting resistance, moisture sensitivity, and elastic modulus[43]. Different studies have different proportions of cement and aggregates, where we recognize that a coarse aggregate content of 15% significantly improves the compressive performance of concrete. Furthermore, from the standpoint of benefits to the environment, higher replacement doses might have negative effects[43]. It has also been established that 15% is the ideal content of coarse RCAs in terms of mechanical properties[43]. But aside from RCAs, this indicator isn't applicable to other kinds of RAC. For example, when replacing NAC with electronic plastics, the proportion of NAC must be increased to ensure the overall performance of concrete because of the relatively poor compressive performance of electronic plastics[22]. Furthermore, the quality of RCAs can be greatly enhanced by speeding up the carbonation process, which will require less cement to achieve the same compressive strength[23]. These methods offer fresh perspectives on how to maximize RAC performance while lessening environmental effect.
Lastly, it's important to think about inventory allocation and database improvement. The incompleteness of database data is a common problem in the LCA process of RCAs[7,8,12,46]. Neglecting substances with little effect on the environment is a common tactic, but it is highly subjective and depends largely on the researcher's knowledge of the substance[30]. During the system evaluation, Cao et al.[34], did not take the base plate into account in the construction inventory. When Cantero-Durango et al.[43] conducted a preliminary assessment of production costs, they disregarded fixed costs, variable costs, taxes, and profits. These techniques place an undue emphasis on the researcher's subjective assessment and personal experience, which could skew the evaluation's findings. For example, some research indicates that because the superplasticizers are used so little, they should not be taken into account when assessing their environmental impact[23]. However, another study indicated that external additives could have a significant impact on acidification[35]. This disparity reflects researchers' differing perspectives on the impact of specific substances and highlights the challenges posed by incomplete data. Another approach for addressing data gaps is to obtain missing data through other channels. Some studies create secondary databases by modifying data from the literature[28], while others adjust data in the Ecoinvent database that do not belong to specific regions to make LCA analysis more relevant to regional characteristics[38]. However, the reliability of data obtained using a single-channel modification method is difficult to ensure. To ensure data reliability and improve work efficiency, it is recommended to use a prioritized hierarchical method based on data availability[37]. For example, different scenarios (such as French, European, and global scenarios) can be used to determine data priorities. Global scenario data can be used with substances that have a low environmental impact. National scenario data should be prioritized for substances with potentially significant impacts. If the scenario data for key substances (such as cement) does not match, previous experimental data at the same location or field data collection should be obtained[37]. This approach can guarantee data integrity while cutting down on pointless work and improving the accuracy of RCAs' environmental impact assessment.
5. Environmental Impact Assessment
The environmental evaluation of RAC is primarily concerned with the long-term sustainability of economic, social, and environmental impacts. This process combines evaluation models and indicators to classify and summarize environmental impacts, providing relevant data to support the life cycle interpretation stage[40]. It intends to determine the potential impact levels caused by various environmental issues (such as ecological balance, human health, environmental safety, resource or energy consumption)[16]. LCIA helps researchers understand the potential environmental impacts of a product or service by converting environmental loads (e.g. emissions and resource consumption) into easily comparable scores using characterization factors[47]. In order to assess potential effects on human health, ecosystem diversity, and resource availability, the assessment for RCAs typically focuses on ten impact categories, i.e., global warming potential (GWP), abiotic depletion potential (ADP), eutrophication potential (EP), ozone depletion, photochemical smog formation, ecotoxicity, fossil fuel depletion, human health particulate matter, human health non-cancer, and human health cancer[36]. Numerous research studies indicate that the usage of NCAs and RCAs significantly impacts the environment, particularly in exacerbating the GWP[9,48-50]. However, besides GWP, existing research generally gives insufficient attention to the human toxicity impacts of RCAs, often providing only brief overviews[3,11,13]. According to research, NCAs play an important role in assessing human toxicity impacts. For example, in the terrestrial ecological toxicity impact category, NCAs contribute at least 9-21% of emissions[1]. Using RCAs can increase human toxicity by over 1% when compared to natural aggregates, according to another study[2]. This suggests that not all aspects of the environmental impact of using RCAs are favorable. Furthermore, differences in environmental conditions, processing technologies, and chemical compositions of natural aggregates between quarries reduce LCIA accuracy significantly. Therefore, to improve the data on cement aggregates in the database, we therefore advise choosing quarries globally that are representative of the geological environment and technical processes. This will offer precise direction for the future growth of LCA databases.
To assess the overall impact of various infrastructures, impact indicators must be normalized and weighted. Normalization connects results to global impacts by multiplying them by global factors in each category. This method makes it easier to forecast the impact of RAC on environmental issues of public concern, such as those addressed by ReCiPe's three core areas: human health, ecosystem quality, and resource scarcity. The European standard EN 15804 takes into account over 15 indicators, including inventory flow and midpoint indicators such as GWP[37]. There could be more indicators added to give an even more comprehensive explanation. Wang et al.[50], included recycled fine powder in their study, broadening the scope of research and incorporating more environmental impact indicators. Environmental impact assessment analysis must take into account multiple impact categories and evaluation techniques, taking into account the ideal water-binder ratio[18,41] and the effect of RCAs on the mechanical properties and durability of RAC[38]. Furthermore, by weighing each equivalent in accordance with predetermined formulas, the LCA assesses the effects on the environment[18]. To ensure the accuracy, comparability, and objectivity of assessment results, weighting and grading methods can be used to quantify RAC's environmental performance. For the purpose of normalizing environmental impact, Pu et al.[23] employed a willingness-to-pay based weighting method. This method achieves comparability and accuracy in assessment results by grading and weighting various impact factors according to their importance and weight distribution. This provides a scientific basis for improving the environmental performance of RAC[16]. Taking into account several impact parameters, however, could increase the error range and decrease the accuracy of the analysis's findings. Ensuring equal precision in evaluation results is heavily dependent on the accuracy of the parameters used by the researchers. As a result, we do not recommend taking into account too many cement properties (such as cement soundness, alkali content, and loss on ignition) during LCA analysis to avoid introducing numerous uncertain factors that could affect the accuracy of the primary environmental impact results.
EDIP 97, EDIP 2003, Eco-indicator 99, IPCC 2001, Environmental Design of Industrial Products 1997, CML 2001, Ecological Scarcity 1997, ReCiPe, and IMPACT 2002+ are some of the mainstream techniques for LCA of RAC in academic research[42]. LCIA methods include problem-oriented midpoint and damage-oriented endpoint approaches. The former transforms environmental impacts into tangible phenomena like GWP, AD and EP, which can be measured with CML2001, ReCiPe, EDIP2003, and IMPACT2002+. The latter converts midpoint impacts into environmental, climate change, human, and resource damages, which can be assessed with ReCiPe, Eco-indicator 99, and IMPACT2002+. A review of the existing literature reveals that the LCIA process for RCAs typically uses the midpoint method for analysis. To provide a more comprehensive assessment of the global issues associated with RCAs, some studies use the endpoint method to improve their results[47,51,52]. When assessing the effects on the environment, these two approaches work well together[47]. Currently, many scholars have conducted LCIA on RAC, yielding comprehensive research findings. Notably, some studies add Economic Input-Output LCA and Life Cycle Cost owing to differing focuses[41,45,53]. Additional techniques like the Rank - Sum Ratio and the Technique for Order Preference by Similarity to Ideal Solution are used to measure compressive strength when evaluating the safety or quality of RAC[42].
The CML baseline method and TRACI method are most frequently used, according to a summary of the literature, to assess the midpoint environmental impacts. The LCIA of RAC is based on general environmental impact assessment models that do not consider regional heterogeneity, with GWP as the main impact category[39], followed by EP. The comparison of NCAs and RCAs in terms of EP takes into account NCA mining locations and transportation distances. Transportation is the second most significant environmental impact factor[54]. Fuel consumption varies by region based on vehicle type and road conditions, and different studies place varying emphasis on raw material transportation. Regional heterogeneity must be taken into account, which will undoubtedly make the adaptability of LCIA model for RAC more difficult. Furthermore, assessing only the environmental benefits of RCAs is insufficient. According to Kleijer et al.[44], changing the replacement rate of RCAs does not accurately reflect market realities. Combining economic and social benefits is necessary for a comprehensive evaluation of RCA's advantages, and many studies integrate life cycle cost analysis for comprehensive assessments[14,55]. Polo-Mendoz et al.[14], used mathematical models such as multiple linear regression, artificial neural networks, and genetic algorithms to achieve more accurate results. This suggests that there is still significant room for improvement in the precision of RCA's single evaluation methods.
6. Life Cycle Interpretation
The interpretation phase, which is the final stage of LCA, consists of summarizing and synthesizing LCI and impact assessment to form conclusions and recommendations[42]. As previously stated, differences in scope and impact assessment methods can influence conclusions, requiring researchers to interpret the final conclusions and assess the data's completeness and representativeness. Furthermore, the information provided in earlier phases (e.g., goal and scope definition, LCI analysis, LCIA) must be identified, quantified, verified, and evaluated in order to draw evaluative conclusions and suggest process improvements that will improve overall environmental benefits.
In recent years, the environmental impact categories included in RCA's LCA results have grown in complexity, increasing the time and calculation methods required. We will not be able to observe all of RCA's environmental impacts if the data, time, models, and resources are inadequate to support the analysis of those impacts, as was covered in the previous section on analytical methods. Including additional environmental impact categories may make LCA results less valuable and credible. As a result, sensitivity and uncertainty analysis have become critical steps in LCA research.
Sensitivity analysis and Monte Carlo simulations become essential when modeling lacks sufficient or trustworthy data to accurately input certain inventory variables and the uncertainties of these variables greatly impact the outcomes of environmental evaluations. Sensitivity analysis seeks to quantify the degree to which particular variables affect the analysis results by demonstrating how LCA results change with changes in the variables under evaluation[54]. The quantity of NAC or RAC used and the distance of transportation are generally regarded as two important variables in LCA of RAC[35,56]. Because cement represents the primary environmental burden in various impact categories within the concrete system, Jiménez et al.[1] chose cement for sensitivity analysis, which revealed that cement type has a significant impact on GWP. According to Zhang et al.[19], the environmental impact of different aggregate transportation methods, such as road, rail, and water, varies significantly. Pu et al.[23] also noted that the main factors causing the environmental impact of RAC are cement production and transportation. Furthermore, Pu et al.[23] investigated the critical transportation distance of RCAs using sensitivity analysis results[23]. It is important to note that transportation distance in LCA has both hypothetical and idealistic characteristics. Ding et al.[27] stated that total transportation is an important factor in determining the environmental impact of NAC and RAC projects. However, due to the localized nature of concrete production, the total transportation distances of different projects vary significantly, necessitating research into the perceived impact of total transportation distances across regions[27]. Pradhan et al.[33] stated that the environmental impact assessment of fixed transportation schemes limited the research's applicability. It is worth noting that some researchers underestimated the transportation variable or made assumptions that lacked practical credibility. Although some studies acknowledged the importance of transportation in impact assessment, they chose to ignore portions of the transportation distance[22], and some studies even excluded the environmental impact associated with the transportation of recycled materials from system boundaries using cut-off methods[28]. To simplify the analysis, some studies assumed that NAC is manufactured and used on-site, with zero transportation distance[42]. Ignoring the transportation distance of equipment used to produce NAC may affect the accuracy of evaluation results. In order to guarantee the precision and dependability of the environmental impact assessment of RAC, we suggest implementing Pu et al.[23] methodology, which entails the explicit classification of the different kinds of transport vehicles and the description of the gasoline types utilized. Furthermore, the analysis was segmented by Pu et al.[23] according to the varying distances between NCAs and urban areas. This improves the overall accuracy of the environmental impact assessment. In conclusion, it is critical to conduct a thorough investigation into relevant factors influencing processes for which data is difficult to obtain, in order to ensure the credibility of the evaluation results.
Monte Carlo simulation is a method of assessing data quality. It entails conducting a large number of random experiments based on the uncertainties of the factors to be studied, estimating the possible distribution of outcomes, and assisting in the prediction and analysis of results under various scenarios[57]. The key points of Monte Carlo simulation are similar to those of sensitivity analysis, requiring careful variable selection. For example, some studies do not consider the environmental impact of high-efficiency water reducers because they are rarely used[23]; or the additives used in RAC preparation are not available in existing databases[33]. In fact, these additives have the potential to increase environmental burdens[35]. Therefore, combining Monte Carlo simulation outputs yields more credible probabilistic evaluation results[30]. However, comparing differences between multiple processes is time-consuming, evaluating the entire LCA (including system boundaries, LCI, and LCIA) is uncommon, and determining the contribution of each parameter to overall uncertainty is difficult[20]. Wang et al.[58] proposed a method for developing a log-normal distribution-based life cycle fundamental function evolution model, resulting in the following analytical models for single and multiple process uncertainty analysis, as shown in Eq. 1 and Eq. 2.
where, Si and GSDi2 are the sensitivity and data quality indicators for processes or substances, respectively.
where, SAi, SBj, GSDAi and GSDBi are the relative sensitivities and corresponding data quality indicators of the unrelated units of processes A and B, respectively. SAk and SBk are the relative sensitivities of the related units of processes A and B, and GSDxk is the corresponding data quality indicator. These two analytical models overcome the limitations of traditional Monte Carlo uncertainty analysis models in LCA (namely complexity, coverage gaps, and difficulty identifying key factors), allowing for objective and scientific energy-saving and emission-reduction evaluations, recommendations, and guidelines[58].
To ensure that LCA results are accurate and reliable, a thorough understanding of system boundaries, functional units, data sources, and allocation principles is required. However, result interpretation in practical applications has limitations, primarily due to regional standard value differences. Different studies frequently disagree in defining boundaries and time frames. A Melbourne study on cement's carbon capture capacity argued that, in addition to the primary 100-year service life examined in mainstream research, an additional 30-year secondary service life should be considered[45]. Because studies have shown that RAC captures significantly more CO2 during secondary use, which is typically not included in building concrete carbon footprint LCA estimates[45]. Furthermore, research on RCA's potential environmental impacts in Chinese concrete production did not use the mainstream "cradle-to-gate" approach, but rather used the "cradle-to-cradle" theory to conduct a closed-loop LCA of RAC utilization in China[46]. The cement used in comparative experiments has different environmental impacts due to varying national standards, as the water-binder ratio frequently varies[48]. Relevant Portuguese research reveals significant environmental impact differences due to varying water-binder ratios[49]. Furthermore, because RCA research must account for volume, strength, and durability, functional unit selection varies across studies[50]. These phenomena are primarily caused by differing understandings of system boundaries and a lack of unified analysis and evaluation standards. The lack of a unified standard system also makes it difficult to compare and verify cement result interpretations, resulting in significant differences between experimental results.
In terms of definition, a review of existing research shows that the first life cycle of RAC is typically set at 100 years[7,16,35]. Carbonation can be almost nonexistent during cement's primary service life[7]. However, studies show that RAC captures significantly more CO2 during its secondary use, as broken-down RCA has a larger surface area exposed to CO2 and carbonates than buildings made of RCAs[7]. It is critical to standardize the analysis period for cement's second life cycle. This factor is frequently excluded from LCA estimates of the carbon footprint of building concrete[7]. However, the duration of the second life cycle has a significant impact on RCA's GWP. Researchers define cement's regeneration phase, or second lifecycle, in three distinct ways: 60 years[18], 30 years[7,54], and 15 years[34]. These differences in the second life cycle period cause varying carbonation results, lowering the credibility of RAC's GWP analysis. Additionally, some authors propose improving predictive models by incorporating carbonation data from RAC used in a variety of secondary life applications[7]. As a result, researchers must concentrate on RCA's carbonation effect in the LCA of RAC. Future research should focus on how cement's carbonation during its second life cycle and the analysis phase affects LCA outcomes.
7. Limitations
We examined the four stages of LCA from a unique perspective not found in other studies. This method discussed data errors at each stage and identified areas for future database improvements. Future research should look into different LCA cases for low-carbon cement to make the results more representative. Furthermore, the current issues and solutions for each component of low-carbon cement LCA should be considered in the context of practical situations, such as national or regional policies and religious beliefs.
8. Conclusions and Discussion
This paper describes the mainstream research methods, evaluates the shortcomings of previous studies, and summarizes the research steps of the LCA of RCAs. The purpose of this study is to pinpoint the divisive and skewed issues that arise in the technical stages of the LCA of RCAs. This study identifies approaches from the literature that can help with these problems or lessen their impact. Additionally, we indicate possible future directions for technological progress in the environmental impact assessment of RCAs and offer guidance for the creation and improvement of relevant databases. The innovation of this paper lies in the novel perspective of reviewing through the four steps of LCA. The explanation of the four steps' fundamental theories comes first, and then the main methods of RCAs research are outlined. The various approaches taken by previous researches to the four aspects of the LCA of RCAs (i.e., goal and scope definition, LCI analysis, LCIA, and interpretation) are then analyzed. Ultimately, the best approaches are chosen and improved.
1) Goal and Scope Definition: Currently, the evaluation process's goal and scope definitions, as well as the functional units, overlook concrete strength and durability, which may result in biased results. As a result, when defining functional units, performance characteristics such as strength, durability, and strength reliability should be considered in addition to volume. The functional units chosen should meet the cement properties requirements specified in the research direction while accurately reflecting environmental impacts. Notably, differences in system boundary settings can result in omissions in certain stages of RAC production and use, resulting in varying environmental impact assessment results.
2) LCI: The LCI process still requires improvement. First, determining transportation distance is critical but difficult to do with high accuracy. Some studies simplify calculations using unsupported assumptions, lowering the credibility of the results. Future research should thoroughly consider transportation tools, fuel types, and trip counts, assigning weights based on statistical probabilities of various scenarios and calculating representative data to accurately reflect the impact of transportation factors. Second, the ratio of RAC to NAC is critical. Increasing the coarse aggregate content can significantly improve compressive strength, but excessive substitution can backfire. The recommended addition amount for coarse aggregate is 15%, taking into account both environmental benefits and mechanical properties. Furthermore, accelerating the carbonation process can significantly improve the quality of RCAs while reducing reliance on NCAs, resulting in increased material efficiency while maintaining equivalent compressive strength. In order to resolve the issue of missing data in the LCA process for RCAs, the database should be improved. One strategy is to ignore substances with minor effects, but this requires researchers to understand the substance, making it subjective. Another approach is to obtain missing data through other channels; however, relying on a single source to modify database data can be untrustworthy. We recommend using a priority-based grading system. If key materials, such as cement, do not match the scenario level, prior experimental or field data collection is required.
3) Environmental Impact Assessment: Existing research has not adequately addressed the human toxicity impact of RCAs, lowering the accuracy of LCIA. We propose that future studies choose globally representative quarries to improve the database and indicate the direction for LCA database expansion. Because too many elements can affect analysis accuracy, it is not recommended to include excessive cement properties in the LCA process in order to avoid lowering the quality of environmental impact results. Furthermore, evaluating the environmental benefits of RCAs alone is insufficient; a comprehensive assessment must take into account economic and social benefits.
4) Life Cycle Interpretation: Transportation distance in the LCA has both hypothetical and idealistic characteristics. For processes with difficult data collection, a thorough investigation of relevant factors is required. Monte Carlo simulation can produce more credible probabilistic evaluation results for the life cycle environmental impact, but it is inefficient when comparing multiple processes. Because of its limited role in overall LCA evaluation, it is difficult to assess each parameter's contribution to overall uncertainty. We recommend that you use the lognormal distribution-based the life cycle fundamental function evolution method. Furthermore, interpretation of cement results is complicated by difficulties in comparative validation and significant biases in experimental results. The analysis period for the second the life cycle of cement should be standardized, and the carbonation effect of RCAs in the LC of RAC should be highlighted. To improve predictive models, it is recommended to include carbonation data from RAC. Future research should investigate the impact of the carbonation effect and the analysis period of cement's second the life cycle on LCA outcomes.
Authors contribution
Zhang J: Writing-original draft, investigation, conceptualization.
Huang Z: Software, methodology.
Zhu Z: Data curation, formal analysis.
Yang S: Visualization.
Wei J: Funding acquisition, supervision, writing-review & editing.
Conflicts of interest
Junxiao Wei is the Editorial Board Member of the journal and other authors declare no conflicts of interest.
Ethical approval
Not applicable.
Consent to participate
Not applicable.
Consent to publication
Not applicable.
Availability of data and material
Not applicable.
Funding
This study was supported by the Program for scientific research start-up funds of Guangdong Ocean University (060302122305).
Copyright
© The Author(s) 2024.
References
-
1. Jiménez C, Barra M, Josa A, Valls S. LCA of recycled and conventional concretes designed using the Equivalent Mortar Volume and classic methods. Constr Build Mater. 2015;84:245-252.
[DOI] -
2. Sereewatthanawut I, Prasittisopin L. Environmental evaluation of pavement system incorporating recycled concrete aggregate. Int J Pavement Res Technol. 2020;13(5):455-465.
[DOI] -
3. Xing W, Tam VWY, Le KN, Hao JL, Wang J. Life cycle assessment of recycled aggregate concrete on its environmental impacts: a critical review. Constr Build Mater. 2022;317:125950.
[DOI] -
4. Wang Z, Zhang Z, Jin X. A study on the spatial network characteristics and effects of CDW generation in China. Waste Manage. 2021;128:179-188.
[DOI] -
5. Huang B, Gao X, Xu X, Song J, Geng Y, Sarkis J, et al. A Life Cycle Thinking Framework to Mitigate the Environmental Impact of Building Materials. One Earth. 2020;3(5):564-573.
[DOI] -
6. Xin G. Review: Development Trends in the Reuse of Waste Materials in Concrete Production. Sci Technol. 2023;8(2):26-30.
[DOI] -
7. Collins F. Inclusion of carbonation during the life cycle of built and recycled concrete: influence on their carbon footprint. Int J Life Cycle Assess. 2010;15(6):549-556.
[DOI] -
8. Shi X, Mukhopadhyay A, Zollinger D, Grasley Z. Economic input-output life cycle assessment of concrete pavement containing recycled concrete aggregate. J Cleaner Prod. 2019;225:414-425.
[DOI] -
9. Yuan H, Zhu L, Zhang M, Wang X. Mechanical behavior and environmental assessment of steel-bars truss slab using steel fiber-reinforced recycled concrete. J Build Eng. 2023;69:106252.
[DOI] -
10. Chen X, Ai Y, Cheng S, Xu X. Performance indexes distribution and correlation analysis of recycled coarse aggregate. J Archit Civ Eng. 2023;40(2):1-10. Chinese.
[DOI] -
11. Tribout C, Escadeillas G, Hodroj M, Nicolas JL, Bergès T. Methodological approach based on life cycle assessment for upcycling leftover concrete into dry industrial mortars. J Build Eng. 2024;86:108868.
[DOI] -
12. Knoeri C, Sanyé-Mengual E, Althaus HJ. Comparative LCA of recycled and conventional concrete for structural applications. Int J Life Cycle Assess. 2013;18(5):909-918.
[DOI] -
13. Abed M, Shmlls M. Analysis of three generations of recycled concrete: an approach using LCA and weighted sum model. Mater Today: Proc. 2023.
[DOI] -
14. Polo-Mendoza R, Martinez-Arguelles G, Peñabaena-Niebles R. Environmental optimization of warm mix asphalt (WMA) design with recycled concrete aggregates (RCA) inclusion through artificial intelligence (AI) techniques. Results Eng. 2023;17(100984):1-15.
[DOI] -
15. Chen D, Chen M, Sun Y, Shaopeng W, Xintao Z, Chen W. Sustainable use of recycled cement concrete with gradation carbonation in artificial stone: Preparation and characterization. Constr Build Mater. 2023;364:129867.
[DOI] -
16. Younis A, Ebead U, Judd S. Life cycle cost analysis of structural concrete using seawater, recycled concrete aggregate, and GFRP reinforcement. Constr Build Mater. 2018;175:152-160.
[DOI] -
17. Vega A DL, Gilberto MA, dos Santos JMO. Life Cycle Assessment of Warm Mix Asphalt with Recycled Concrete Aggregate. IOP Conf Ser: Mater Sci Eng. 2019;603(5):052016.
[DOI] -
18. Abushanab A, Alnahhal W. Life cycle cost analysis of sustainable reinforced concrete buildings with treated wastewater, recycled concrete aggregates, and fly ash. Results Eng. 2023;20:101565.
[DOI] -
19. Zhang Y, Luo W, Wang J, Wang Y, Xu Y, Xiao J. A review of life cycle assessment of recycled aggregate concrete. Constr Build Mater. 2019;209:115-125.
[DOI] -
20. Colangelo F, Forcina A, Farina I, Petrillo A. Life Cycle Assessment (LCA) of Different Kinds of Concrete Containing Waste for Sustainable Construction. Building. 2018;8(5):70.
[DOI] -
21. Colangelo F, Petrillo A, Farina I. Comparative environmental evaluation of recycled aggregates from construction and demolition wastes in Italy. Sci Total Environ. 2021;798:149250.
[DOI] -
22. Goh PG, Maghfouri M, Onn CC, Loo SC. Life cycle assessment on recycled e-waste concrete. Case Stud Constr Mater. 2022;17:e01412.
[DOI] -
23. Pu Y, Li L, Shi X, Wang Q, Abomohra A. A comparative life cycle assessment on recycled concrete aggregates modified by accelerated carbonation treatment and traditional methods. Waste Manage. 2023;172:235-244.
[DOI] -
24. Thwe E, Khatiwada D, Gasparatos A. Life cycle assessment of a cement plant in Naypyitaw, Myanmar. Cleaner Environ Syst. 2021;2(12):100007.
[DOI] -
25. Ige OE, Olanrewaju OA, Duffy KJ, Obiora C. A review of the effectiveness of Life Cycle Assessment for gauging environmental impacts from cement production. J Cleaner Prod. 2021;324:129213.
[DOI] -
26. Li G, Guo XL, She AM. Study on environmental impact of recycled concrete with limestone powder based on whole life cycle assessment. China Concr Cem Prod. 2023;(12):79-88. Chinese.
[DOI] -
27. Ding T, Xiao J, Tam VWY. A closed-loop life cycle assessment of recycled aggregate concrete utilization in China. Waste Manage. 2016;56:367-375.
[DOI] -
28. Vega-Araujo D, Martinez-Arguelles G, Santos J. Comparative life cycle assessment of warm mix asphalt with recycled concrete aggregates: A Colombian case study. Procedia CIRP. 2020;90(5):285-290.
[DOI] -
29. Pesta J, Pavlo T, Fortova K, Repka J. Recycled concrete for foundation structure: LCA case study. IOP Conf Ser: Mater Sci Eng. 2021;1196(1):012012.
[DOI] -
30. International Organization for Standardization. ISO 14040:2006 : Environmental management-life cycle assessment-principles and framework: International Organization for Standardization; 2006. Available from: https://www.iso.org/standard/37456.html
-
31. Xie K. The environmental load research of construction waste recycling [dissertation]. Beijing: Beijing University of Technology; 2013. Chinese.
-
32. Zhu L, Su Q, Yan RS, Liu ZL, Ren BJ, Tao CX, et al. Theory and prospect of cement life cycle evaluation for environmental impact. Cement. 2023;5:16-22. Chinese.
[DOI] -
33. Pradhan S, Tiwari BR, Kumar S, Barai SV. Comparative LCA of recycled and natural aggregate concrete using Particle Packing Method and conventional method of design mix. J Cleaner Prod. 2019;228:679-691.
[DOI] -
34. Cao Z, Zhou L, Gao Z, Huang Z, Jiao X, Zhang Z, et al. Comprehensive benefits assessment of using recycled concrete aggregates as the substrate in constructed wetland polishing effluent from wastewater treatment plant. J Cleaner Prod. 2021;288:125551.
[DOI] -
35. Serres N, Braymand S, Feugeas F. Environmental evaluation of concrete made from recycled concrete aggregate implementing life cycle assessment. J Build Eng. 2016;5:24-33.
[DOI] -
36. Kurda R, Silvestre JD, de Brito J. Life cycle assessment of concrete made with high volume of recycled concrete aggregates and fly ash. Resour Conserv Recycl. 2018;139:407-417.
[DOI] -
37. Hube S, Zaqout T, Ögmundarson Ó, Andradóttir HÓ, Wu B. Constructed wetlands with recycled concrete for wastewater treatment in cold climate: Performance and life cycle assessment. Sci Total Environ. 2023;904:166778.
[DOI] -
38. Nwakaire CM, Onn CC, Yap SP, Yuen CW, Koting S, Mo KH, et al. The strength and environmental performance of asphalt mixtures with recycled concrete aggregates. Transp Res Part D: Transp Environ. 2021;100:103065.
[DOI] -
39. Kurda R, Silvestre JD, de Brito J, Ahmed H. Optimizing recycled concrete containing high volume of fly ash in terms of the embodied energy and chloride ion resistance. J Cleaner Prod. 2018;194:735-750.
[DOI] -
40. Mostert C, Sameer H, Glanz D, Bringezu S. Climate and resource footprint assessment and visualization of recycled concrete for circular economy. Resour Conserv Recycl. 2021;174:105767.
[DOI] -
41. Pavlu T, Pesta J, Vlach T, Fortova K. Environmental Impact of Concrete Slab Made of Recycled Aggregate Concrete Based on Limit States of Load-Bearing Capacity and Serviceability-LCA Case Study. Materials. 2023;16(2):616.
[DOI] -
42. Deng Y, Zhang K, Fu Y, Huiling Z, Zhigang Y. Analysis and optimization of design parameters for recycled concrete modified with nano-CaCO3 considering environmental and economic and mechanical properties. J Mater Cycles Waste Manage. 2023;25(6):3651-3663.
[DOI] -
43. Cantero-Durango J, Polo-Mendoza R, Martinez-Arguelles G, Fuentes L. Properties of Hot Mix Asphalt (HMA) with Several Contents of Recycled Concrete Aggregate (RCA). Infrastruct. 2023;8(7):109.
[DOI] -
44. Kleijer AL, Lasvaux S, Citherlet S, Viviani M. Product-specific Life Cycle Assessment of ready mix concrete: Comparison between a recycled and an ordinary concrete. Resour Conserv Recycl. 2017;122:210-218.
[DOI] -
45. Guo Z, Tu A, Chen C, Lehman DE. Mechanical properties, durability, and life-cycle assessment of concrete building blocks incorporating recycled concrete aggregates. J Cleaner Prod. 2018;199:136-149.
[DOI] -
46. Luo W, Liu S, Hu Y, Hu D, Kow KW, Pang C, et al. Sustainable reuse of excavated soil and recycled concrete aggregate in manufacturing concrete blocks. Constr Build Mater. 2022;342:127917.
[DOI] -
47. Huijbregts MAJ, Steinmann ZJN, Elshout PMF, Stam G, Verones F, Vieira M, et al. ReCiPe2016: a harmonised life cycle impact assessment method at midpoint and endpoint level. Int J Life Cycle Assess. 2016;22(2):138-147.
[DOI] -
48. Kul A, Ozel BF, Ozcelikci E, Gunal MF, Ulugol H, Yildirim G, et al. Characterization and life cycle assessment of geopolymer mortars with masonry units and recycled concrete aggregates assorted from construction and demolition waste. J Build Eng. 2023;78(1):107546.
[DOI] -
49. Paula Junior AC, Jacinto C, Oliveira T, Gunal MF, Ulugol H, Yildirim G, et al. Characterisation and Life Cycle Assessment of Pervious Concrete with Recycled Concrete Aggregates. Crystals. 2021;11(2):209.
[DOI] -
50. Wang C, Cheng L, Ying Y, Yang FH. Utilization of all components of waste concrete: Recycled aggregate strengthening, recycled fine powder activity, composite recycled concrete and life cycle assessment. J Build Eng. 2024;82:108255.
[DOI] -
51. Hong J, Shaked S, Rosenbaum RK, Jolliet O. Analytical uncertainty propagation in life cycle inventory and impact assessment: application to an automobile front panel. Int J Life Cycle Assess. 2010;15(5):499-510.
[DOI] -
52. Cheng L, Jin H, Liu J, Xing F. A comprehensive assessment of green concrete incorporated with municipal solid waste incineration bottom: Experiments and life cycle assessment (LCA). Constr Build Mater. 2024;413:134822.
[DOI] -
53. Abed M, Fort J, Rashid K. Multicriterial life cycle assessment of eco-efficient self-compacting concrete modified by waste perlite powder and/or recycled concrete aggregate. Constr Build Mater. 2022;348:128696.
[DOI] -
54. Cassiani J, Martinez-Arguelles G, Peñabaena-Niebles R, Keßler S, Dugarte M. Sustainable concrete formulations to mitigate Alkali-Silica reaction in recycled concrete aggregates (RCA) for concrete infrastructure. Constr Build Mater. 2021;307(124819):124919.
[DOI] -
55. Moro C, Francioso V, Lopez-Arias M, Velay-Lizancos M. CO2 curing of mortar with natural and recycled concrete aggregate: An environmental and economic assessment. Constr Build Mater. 2023;399:132587.
[DOI] -
56. Wang X, Liu Z, Liu C, Wang L, Chen M, Yue GB. Study of mix design and performance of alkali-activated concrete with recycled concrete aggregate. Constr Build Mater. 2023;400:132882.
[DOI] -
57. Ziyadi M, Al-Qadi IL. Model uncertainty analysis using data analytics for life-cycle assessment (LCA) applications. Int J Life Cycle Assess. 2018;24(5):945-959.
[DOI] -
58. Wang Y, Hong J, Wang F, Sun MX. The development of life cycle assessment theory research in China and analysis of countermeasure. Acta Ecol Sin. 2016;36(22):7179-7184. Chinese.
[DOI]
Copyright
© The Author(s) 2024. This is an Open Access article licensed under a Creative Commons Attribution 4.0 International License (https://creativecommons.org/licenses/by/4.0/), which permits unrestricted use, sharing, adaptation, distribution and reproduction in any medium or format, for any purpose, even commercially, as long as you give appropriate credit to the original author(s) and the source, provide a link to the Creative Commons license, and indicate if changes were made.
Publisher’s Note
Share And Cite