Abstract
Over the past two decades, the field of robotics in construction has evolved into an interdisciplinary research domain that combines a multitude of pressing technologies. This work introduces an innovative review framework that assesses the interconnection between robotics in construction and automation, while also examining advancements in technologies. In this research, a novel classification framework was created and a comprehensive literature review was performed to shed light on recent developments in the field of robotic construction. The objectives are to delineate the diverse dimensions of robotics in construction, uncover the underlying themes and sub-themes within these dimensions, identify key research gaps in the current studies, and provide recommendations for future research endeavors. This paper concluded that the existing research focus primarily on technical aspects in robotics within construction, neglecting environmental considerations, while identifying a lack of long-term studies on structural performance, hindering concerns about durability. Additionally, challenges persist in integrating robotics into construction workflows without disruption, compounded by the absence of standardized practices and regulations, alongside concerns about safe human-robot interaction, affordability, and accessibility. Furthermore, inadequate training programs for workers and ethical concerns regarding job displacement, privacy, and societal impacts underscore the need for careful examination in the responsible and ethical deployment of robotics technologies. Finally, research efforts should emphasize the development of user-friendly interfaces and ergonomic designs for construction robots to enhance their usability and acceptance among workers, ultimately contributing to the successful integration of robotics into construction practices.
Keywords
1. Introduction
Robotics in construction (RiC) is a field of technology and engineering that involves the use of robotic systems and automation in various aspects of the construction industry[1]. From 1978 to 1988, significant strides were made in developing construction robots to augment efficiency and tackle prevalent challenges within the construction sector. Notably, the Japanese general contractor Shimizu Corporation pioneered the creation of the first on-site construction robots in response to a scarcity of skilled labor, subpar construction quality, and negative public perception[2]. This era saw the emergence of approximately 50 distinct construction robot systems[3]. Additionally, a pivotal development in 1988 known as robot oriented design (ROD) was introduced to streamline the integration of robotic technology into construction processes by establishing a robot-oriented approach to construction and design[4]. These early achievements in construction robotics laid a foundation for subsequent advancements in the field.
In the ever-evolving realm of construction, technological advancements have ushered in a new era marked by efficiency and precision robotics construction, a cutting-edge field at the intersection of robotics and architecture that promises to reshape how we build the world. By harnessing the power of automation and artificial intelligence (AI), robotic construction presents a paradigm shift in the construction industry, where robots and machines are becoming indispensable tools on construction sites[5-8].
One of the pivotal tools in the arsenal of robotic construction is building information modeling (BIM). BIM is a digital representation of a building's physical and functional characteristics, offering a holistic view of a construction project from inception to completion. When combined with robotic construction techniques, BIM becomes a potent catalyst for innovation, enabling architects, engineers, and builders to optimize the construction process in unprecedented ways[9-12].
Comprehending RiC necessitates a holistic comprehension that considers technological, technical, and environmental constraints, as well as the interplay between these factors. The unique contribution of this study resides in introducing of a classification framework that encompassing these dimensions, including the technological, technical, and environmental aspects of RiC.
The field of RiC is considered dynamic and connected to many other fields, different technologies have emerged and evolved in the field of robotics in construction in the last years[13]. 3D Printing has emerged as a pioneer technology for constructing components on-site. It enables the creation of complicated structures and holds the abilities to reduce material loss, marking an essential advancement in construction industry[14]. Robotic Arms have become pivotal in construction activities like bricklaying, welding, and concrete pumping. These robotic arms enhance construction speed and overall quality as they are precise and consistent, showcasing the potential for increased automation in the industry[15]. Drones are also being employed more to survey, map, and monitor sites. These aerial vehicles boost site control efficiency, enhance safety protocols by providing real-time data[16].
AI is another technology integrated into construction robotics, leading to improvements in decision-making operations and the automation of repetitive tasks[16]. Machine learning (ML) algorithm technology plays a crucial role in analyzing data, contributing to enhancements in project management and implementation[17].
Construction robotics software has become critical in managing and coordinating robotic systems. These new software programs provide features for designing, controlling, and adjusting robotic performance, which enhances operational efficiency[18]. Lastly, augmented reality (AR) and virtual reality (VR) play a fundamental role in design visualization, planning the construction process, and training. These mesmerizing technologies have led to improve communication among project stakeholders and promote effective collaboration, which changed the way of how construction projects are conceptualized and executed[19].
RiC has evolved into a profoundly interdisciplinary research domain that combines robotics with a spectrum of technologies, including additive manufacturing (AM), BIM, and deep learning. Consequently, the body of literature pertaining to RiC is extensive, multifaceted, and fragmented[20]. There are several literature reviews on RiC: some focuses on technical aspects[21-23], and some on the technologies involved[5,22,24]. A comprehensive review on the role of automation and robotic in public-private partnerships (PPP) was also presented[25].
However, it is noteworthy that most of the existing studies primarily represent opportunities and challenges in RiC research without addressing a comprehensive classification framework. The primary contribution of this paper lies in the introduction of a framework, informed by a thorough review of the literature that not only identifies the intricate interactions among various aspects of RiC but also establishes connections between them and the envisioned methodologies, techniques, and challenges in the field.
This paper introduces a systematic flowchart outlining an analytical approach based on bibliometric and bibliographic research analyses concerning RiC. VosViewer software was employed to facilitate the creation of keyword clusters and the identification of the most impactful keyword patterns within each scenario. Additionally, a comprehensive framework is presented that brought to the forefront the significant themes and research categories related to RiC over the recent six years (2018-2023).
This paper develops a classification framework to cover the different aspects associated with the use of robotic technology in the construction industry, thus providing a comprehensive overview of where the research on robotic construction has focused. In order to determine and fulfill the gaps of the research on RiC field, this literature review raised the following questions:
a). Which primary dimensions of RiC does existing research primarily focus on? What specific research themes and subthemes are associated with the identified dimensions in previous studies?
b). What significant gaps exist in the current body of research within the field of robotic construction, and what promising future directions and research horizons can be identified?
The paper is organized as follows: Section 2 illustrates the methodology employed for conducting a comprehensive literature review, providing the outcomes of bibliometric and bibliographic analyses. This section also introduces and expounds upon the classification framework devised in the context of this review. Subsequently, Section 3 is dedicated to addressing the research questions and expounding upon the overarching findings. Lastly, Section 4 proffers a prospective research agenda pertaining to robotic construction, accompanied by conclusive remarks.
2. Materials and Methods
In pursuit of addressing the research questions raised in this study, the authors conducted a systematic literature review, including an examination of technological, technical, and environmental aspects. This approach involved the implementation of bibliometric and bibliographic analyses. The bibliometric analysis delt with identifying a diverse range of topics within the domain of RiC, utilizing citation analysis and keyword clustering techniques. Subsequently, a bibliographic analysis was conducted to qualitatively delve into specific initiatives related to distinct topics by thoroughly reviewing the content of the research papers. Combining these two analytical methods was essential to cultivating a comprehension of the existing literature. Figure 1 represents the sequential stages employed to construct the systematic approach employed in this study. This paper's bibliometric and bibliographic methods form part of the overall review methodology derived from Mayrin. The following are the key steps of the applied methods: • Step 1: Collection of related data; • Step 2: Descriptive analysis of the inspected literature; • Step 3: Introduction of a classification framework that categorizes the examined studies on RiC; • Step 4: Assessment of the materials identified in Step 1, utilizing the classification framework formulated in Step 3.
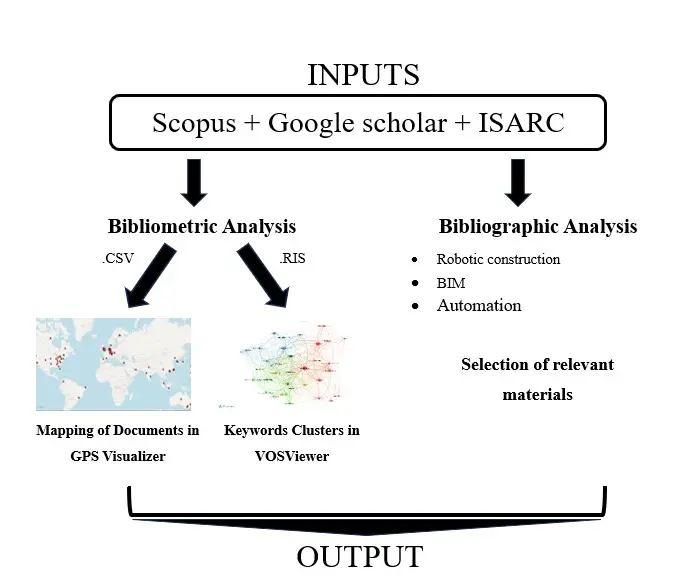
Figure 1. Bibliometric and bibliographic methods applied in the literature review. ISARC: International Symposium on Automation and Robotics in Construction; BIM: building information model; GPS: global positioning system; CSV: comma-separated values; RIS: research information systems.
2.1 Collection of relevant materials
This phase involved collecting a substantial of literature. In order to collect the materials, a set of essential keywords was created to facilitate the identification of related research papers. This preparatory step ensured a methodical search and screening process for published documents that align with the scope of the inquiries presented in the initial section of this article. Three databases were chosen: Elsevier's Scopus, Google Scholar, and International Symposium on Automation and Robotics in Construction (ISARC) Proceedings. Considerations of relevance, comprehensiveness, accessibility, peer-reviewed content, search capabilities, and previous research recommendations meticulously guided the selection of databases for the research. Prioritizing databases specializing in robotics within architectural and engineering construction industries ensured access to scholarly articles and peer-reviewed journals specific to the topic. These selected databases are recognized for their extensive coverage across disciplines, offering a diverse range of studies and publications pertinent to the research. Their widespread use facilitates easy access to a vast collection of literature, while advanced search features enable precise retrieval of relevant research papers, enhancing the efficiency of the research process. However, it's acknowledged that while these databases were chosen for their strengths, they may not encompass all available literature on the subject.
The search phase was done by identifying three main keywords to be used: robotic construction, BIM, and automation. By incorporating these keywords into the literature review, the researchers aim to demonstrate a comprehensive approach to examining the latest research, developments, and trends in the field of robotic construction, encompassing technological advancements, digital tools, and automation strategies. Robotic construction involves the use of robots and automated systems in various construction tasks, offering advantages such as increased productivity, improved safety, and precision. By focusing on this keyword, the researchers aim to explore the latest advancements, applications, and challenges in implementing robotics within the construction industry. BIM is a digital representation of a facility's physical and functional characteristics. It facilitates collaboration, improves decision-making, and enhances efficiency throughout the construction lifecycle. Including this keyword allows to explore how BIM interfaces with robotics and automation in construction. Automation involves the use of technology to perform tasks with minimal human intervention. It is closely related to robotic construction, encompassing various automated processes and systems utilized in construction operations. By incorporating this keyword, the research aims are to explore the intersection between automation and robotics in construction.
Four sets of keywords were developed. Set 1 focused on integrating BIM and RiC and included the following keywords: "robotic construction" and "BIM". The second set combined automation and RiC, and it included the key words "robotic construction" and "automation". The 3rd set involved merging BIM with Automation and the keywords used were "BIM" and "automation". Finally, the 4th set merged the three fields and the keywords "robotic construction", "BIM", and "automation" were used in this set. Initially, a timeline commencing in 2018 was selected with the intention of uncovering the most recent advancements in theory and contributions while emphasizing the subject's relevance over the past six years. Table 1 presents the number of documents retrieved from both databases for each set of keywords.
Database | Number of Retrieved Articles | |||
Set 1 | Set 2 | Set 3 | Set4 | |
Google scholar | 2,680 | 16,900 | 5,350 | 2,420 |
Elsevier's Scopus | 390 | 2,796 | 411 | 152 |
ISARC Proceedings | 538 | 803 | 1,226 | 1,241 |
Total | 3,608 | 20,499 | 6,987 | 3,813 |
ISARC: International Symposium on Automation and Robotics in Construction.
The framework proposed in this research reflects the strong relationship between RiC initiatives, automation, and BIM through bibliometric and bibliographic analyses. The focus for future research was determined following the acquisition of bibliometric results from the five aforementioned searches. The bibliometric analysis was executed employing VosViewer Software, version 1.6.19, developed at Leiden University, Leiden, Netherlands, which seamlessly integrates with the databases utilized in this study. The research outcomes were retrieved in (.ris) format and subsequently imported into the software. Upon the establishment of cluster maps, they subsequently guided the pursuit of three additional types of research, bolstered by the findings derived from the initial five cluster visualizations generated through VosViewer software. The specifics of all the conducted research are outlined as follows:
2.1.1 Elsevier's Scopus
This section describes the steps that were taken to collect the related data from Scopus database based on the chosen keywords, years, language, and type of articles. The number of retrieved documents was also provided for each set of keywords was provided as well.
1. "Robotic construction" and "BIM" were used together in the "title" and "abstract" field. The research was limited to the last six years (2018-2023), and the language was limited to English. Document type was set to review articles and research articles, Subject Areas were limited to engineering, environmental science, energy. A total of 390 documents were retrieved.
2. "Robotic construction" and "automation" were used together in the "title" and "abstract" field. The same filters for years, language, article type, and subject areas as in point 1 were applied. A total of 2,796 documents were retrieved.
3. "BIM" and "automation" were used together in the "title" and "abstract" field. The same filters for years, language, article type, and subject areas as in point 1 were applied. A total of 411 documents were retrieved.
4. "Robotic construction" and "BIM" and "automation" were used together in the "title" and "abstract" field. The same filters for years, language, article type, and subject areas as in point 1 were applied. A total of 152 documents were retrieved.
2.1.2 Google Scholar
This section describes the steps taken to collect the related data from the Google Scholar database based on the chosen keywords, years, language, and type of articles. The number of retrieved documents was also provided for each set of keywords was provided as well.
1. "Robotic construction" and "BIM" were used together in the search field. The research was limited to the last 6 years (2018-2023) and the language was limited to the English language. A total of 2,680 documents were retrieved.
2. "Robotic construction" and "automation" were used together in the search field. The same filters for years and language as in point 1 were applied. A total of 16,900 documents were retrieved.
3. "BIM" and "automation" were used together in the search field. The same filters for years and language as in point 1 were applied. A total of 5,350 documents were retrieved.
4. "Robotic construction" and "BIM" and "automation" were used together in the search field. The same filters for years and language as in point 1 were applied. A total of 2,420 documents were retrieved.
2.1.3 ISARC proceedings
This section describes the steps that were taken to collect the related data from ISARC Proceedings database based on the chosen keywords. The number of retrieved documents was also provided for each set of keywords was provided as well. It should be noting that limiting the search timeline, document type, and subject area was not applicable on the website and the database only provides papers in English Language.
1. "Robotic construction" and "BIM" were used together in the search field. A total of 538 documents were retrieved.
2. "Robotic construction" and "automation" were used together in the search field. A total of 803 documents were retrieved.
3. "BIM" and "automation" were used together in the search field. A total of 1,226 documents were retrieved.
4. "Robotic construction" and "BIM" and "automation" were used together in the search field. A total of 1,241 documents were retrieved.
2.2 Descriptive analysis of the materials
Through the analysis of publication, using the Scopus analysis tools[26], rates within the specified timeframe (2018-2023), Figure 2 illustrates a continuous upward trend in the number of research papers dedicated to RiC. This escalation can be attributed to the escalating interest in RiC over recent years, driven by the increasing fascination with robotic solutions poised to transform the traditional construction sector. The persistent growth rate depicted in Figure 2 reflects the saturation of existing construction methodologies and the pressing demand for modernization, culminating in the pursuit of more resilient and innovative construction practices.
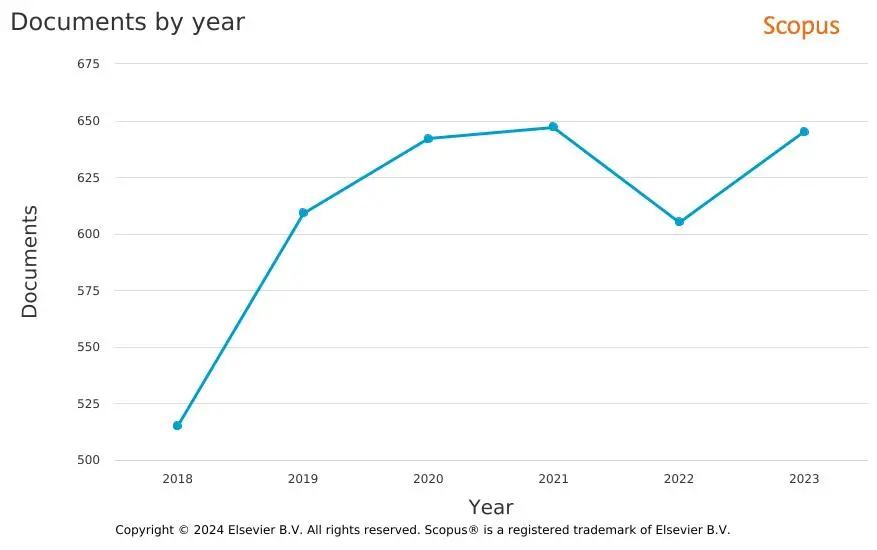
Figure 2. Documents number on Scopus with the keywords "robotic construction", "BIM", and "automation" by year. BIM: building information model.
The papers incorporated into this review were sourced from a diverse array of 26 journals and conference proceedings, representing 42 different countries. Figure 3 visually presents the distribution of analyzed papers across these various origins. While the United States stands out as the primary source of information on the subject, it is noteworthy that China, Germany, and United Kingdom have exhibited notable growth in their research output in recent years. It is essential to highlight that this review exclusively considered papers in the English language, a factor that may have directly influenced the research output observed.
Figure 4 underscores the distribution of analyzed papers across various publishing journals and conference proceedings. At this analytical level, it becomes evident that Proceedings of the International Symposium on Automation and Robotic in Construction has emerged as the primary publication outlet with the highest number of contributions dedicated to RiC, followed by "automation in construction".
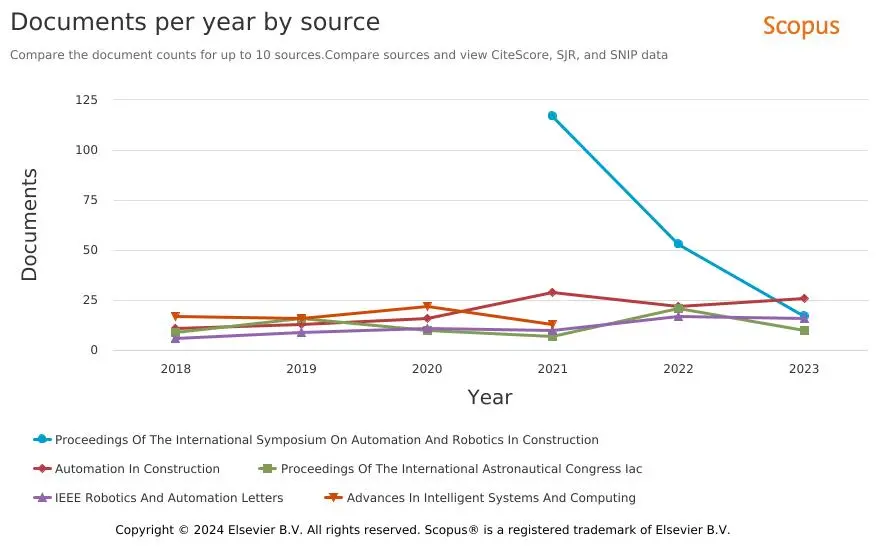
Figure 4. Sources of documents retrieved from Scopus contain the keywords "robotic construction", "BIM", and "automation" from 2018-2023. BIM: building information models.
GPS visualizer online software was used to create a map illustrating the origins of the retrieved documents and their affiliations.
All the citation information from the chosen papers (title, abstract, author, and keywords) were uploaded to VosViewer software to create the keyword clusters. Before this process, the citation information in (.ris) format obtained from Scopus was transformed into localization data, which included latitude, longitude, name, and description fields. This transformation was facilitated through the utilization of BibExcel software, version 1.0. Subsequently, this localized information was integrated into the GPS Visualizer software, which processed and geocoded the data, ultimately generating a map that visually represented the countries of origin for the Scopus documents. Due to Google Scholar's unavailability of citation information documents in the required format for direct integration with BibExcel, the countries of origin for papers from Google Scholar were manually added. Figure 5 visually illustrates the countries of origin for the analyzed documents.
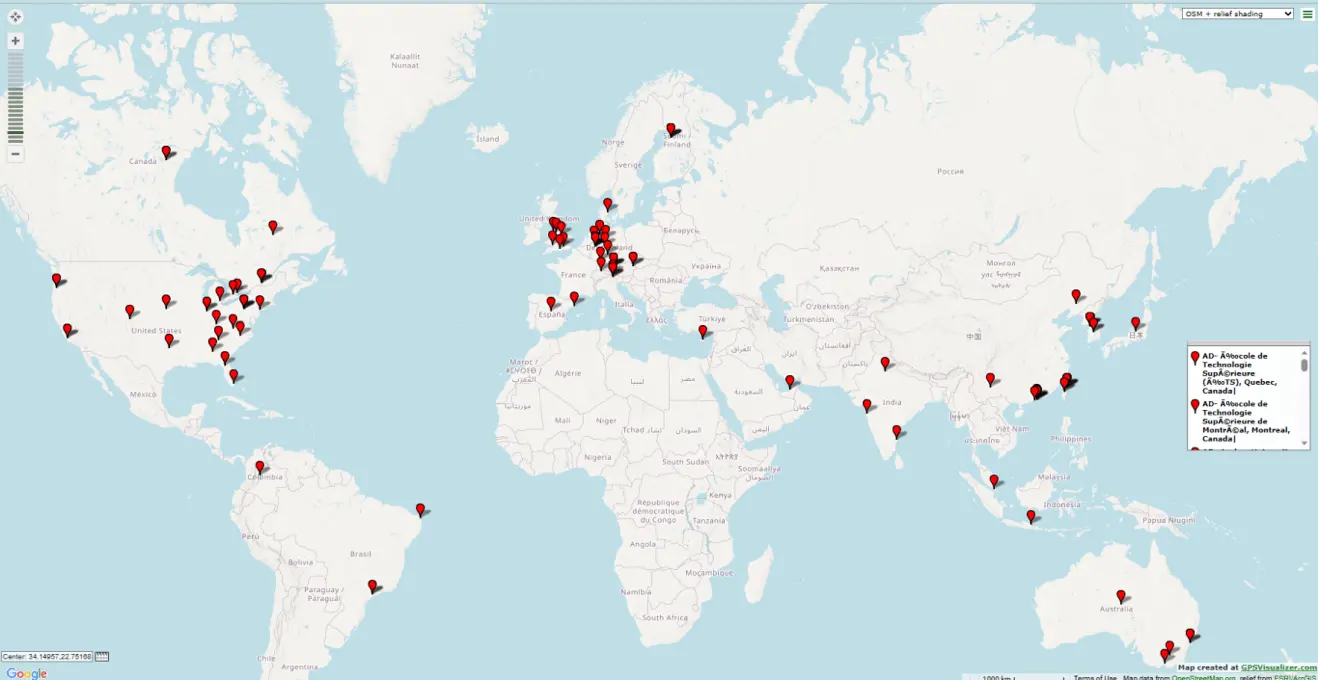
Figure 5. The countries of origin for the analyzed documents from GPS visualizer software. GPS: global positioning system.
The descriptive review conducted in this paper encompassed a bibliometric analysis, which entailed the input of the citation information from the selected articles, including title, abstract, author, and keywords, into the VosViewer software. Subsequently, this process facilitated the creation of keyword clusters. The maps were generated using the binary counting option to eliminate duplicated documents. Following the initial analysis, the VosViewer software determined the frequency of keywords appearing in both the title and abstract fields of all uploaded documents. The number of keywords was significantly reduced by setting a minimum occurrence threshold. Subsequently, a relevance score was computed, and the most fitting terms were subsequently chosen. Ultimately, the list of keywords was revealed. Generic terms unrelated to the subject matter, such as "ease," "time," "place," and "thing," were removed before generating the cluster map. Each generated map underwent thorough examination and served as a tool for refining subsequent research.
The initial search utilized the terms "robotic construction" and "BIM," yielding 390 documents in total. When these (.ris) documents were imported into VosViewer, more than 9,670 keywords were identified within the "title" and "abstract" fields. By imposing a minimum occurrence threshold of 17, only 127 keywords met this criterion. Among these, 75 were considered more relevant, as determined by a relevance calculation within the software itself. Subsequent to verifying the preliminary findings and excluding generic words unrelated to the subject, the initial cluster analysis results were generated, as depicted in Figure 6.
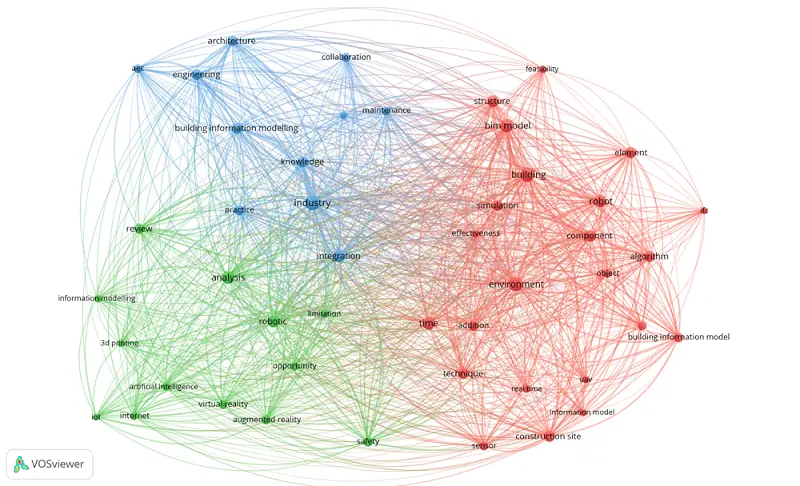
Figure 6. Cluster map generated from the research results using the keywords "robotic" and "BIM". BIM: building information models.
The first and second clusters (highlighted in blue and green) prominently emphasize key aspects within the technological dimension of the RiC concept, including "internet", "artificial intelligence", "IoT", "3D printing", and "digital twin". These keywords serve as valuable tools for refining searches related to the technological facets of RiC. The significance attributed to these terms underscores the substantial role of Information Technology (IT) in the context of RiC. Conversely, the third cluster (in red) underscores technical, environmental, and managerial aspects of the RiC subject, featuring keywords such as "construction site", "environment", "BIM model", and "technique". Collectively, the cluster map illuminates the three primary dimensions prevalent in discussions about RiC: technology, technical, and managerial. It also suggests that while a holistic perspective is essential for a comprehensive understanding of the subject, each dimension warrants specific consideration.
The second search that used the terms "robotic construction" and "automation," resulted in 123 documents in total. When the (.ris) documents were imported into VosViewer, more than 61,016 keywords were identified within the "title" and "abstract" fields. By imposing a minimum occurrence threshold of 90, only 123 keywords met this criterion. Among these, 75 were considered more relevant, as determined by a relevance calculation within the software itself. After verifying the preliminary findings and excluding generic words unrelated to the subject, the initial cluster analysis results were generated, as shown in Figure 7. Similarly, the second search emphasized the significance of IT technologies in RiC literature, particularly the terms "artificial intelligence" and "internet." Additionally, the keyword "robotic process automation" emerged as notably important. As with the first map, this analysis resulted in three clusters centering on a distinct subject dimension.
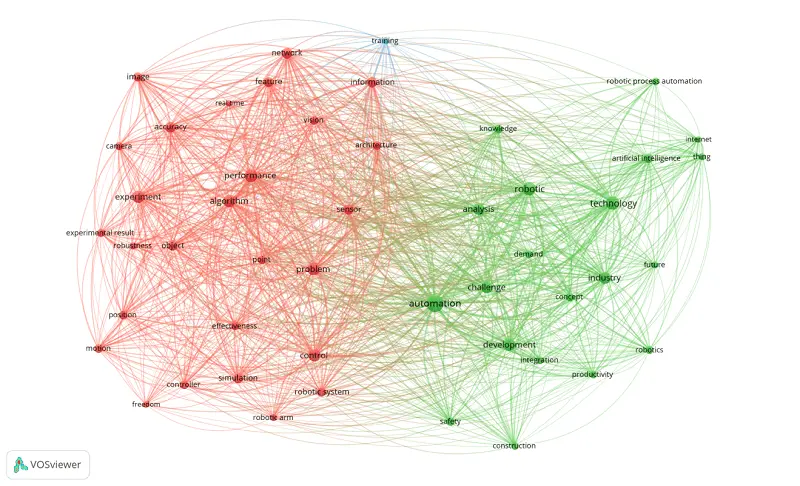
Figure 7. Cluster map generated from the research results using the keywords "robotic" and "BIM". BIM: building information models.
The third search, employing the terms "BIM" and "Automation," retrieved 1,787 documents and revealed more than 10,500 keywords within the "title" and "abstract" fields. By imposing a minimum occurrence threshold of 20, the dataset was refined to 117 keywords, among which 70 were deemed more pertinent. After verifying the preliminary findings, the final clusters are presented in Figure 8. The cluster map generated exhibits three distinct clusters: the red cluster emphasizes the managerial dimension, featuring keywords like "planning", "productivity", "maintenance", and "safety." The green and blue clusters, on the other hand, underscore the technological aspects of robotic in RiC, with keywords such as "point cloud", "digital twin", "algorithm", and "IoT" taking center stage.
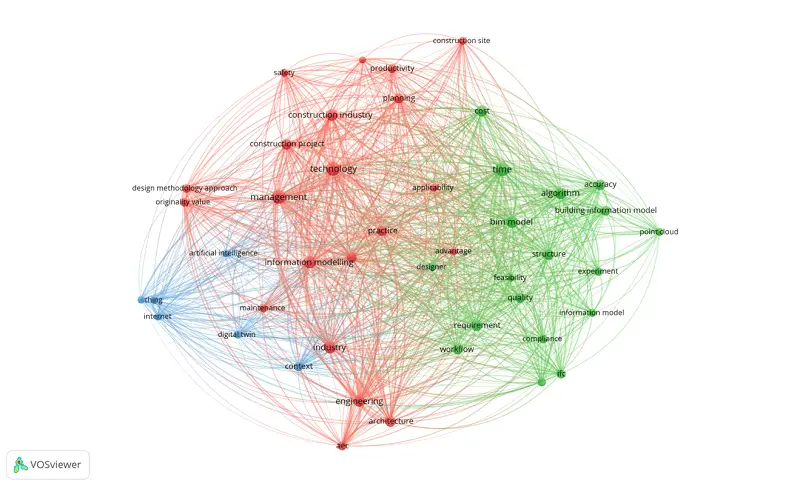
Figure 8. Cluster map generated from the research results using the keywords "BIM" and "automation". BIM: building information models.
The last search, incorporating the terms "robotic," "BIM," and "automation," yielded 55 documents and unveiled more than 3,000 keywords within the "title" and "abstract" fields. Applying a minimum occurrence threshold of 7, the dataset was distilled to 79 keywords, of which 47 were considered more relevant. The ultimate clusters are presented in Figure 9 after validating the preliminary findings. The resulting cluster map features three distinct clusters: the green cluster highlights the managerial dimension, with keywords such as "quality", "accuracy", and "time." In contrast, the red and blue clusters accentuate the technological facets of RiC, showcasing keywords like "virtual reality", "digital twin", "drone", and "IoT" as the focal points.
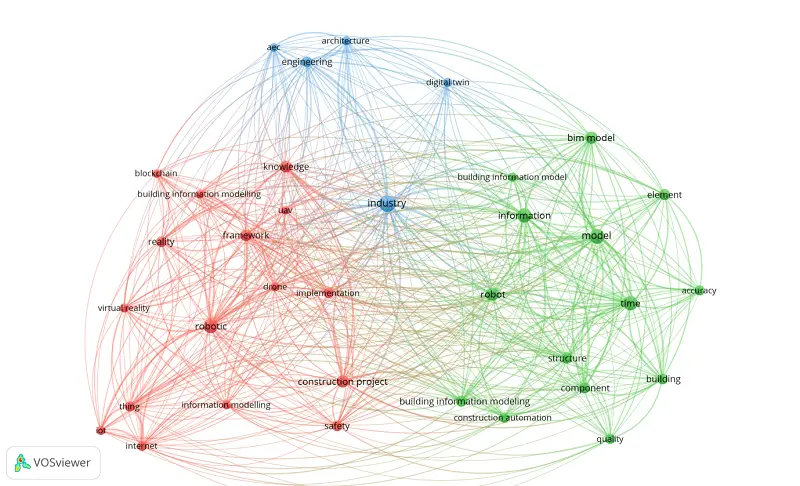
Figure 9. Cluster map generated from the research results using the keywords "robotic" and "BIM" and "automation". BIM: building information models.
2.3 Analysis of papers
In this section, the focus shifted to the clustering of the selected body of work, a process informed by the prior bibliometric analysis conducted. With the retrieval of over 20,000 documents across the initial four types of research, the keyword clusters generated through VosViewer software offered valuable insights into potential refinements within the realm of RiC. Subsequently, four types of research were developed, leveraging these keyword enhancements. This endeavor culminated in the compilation of an extensive corpus comprising more than 7,000 research papers.
Within the collected corpus of literature, a deliberate selection process identified the 25 most pertinent documents from each research cluster, as determined by the bibliometric analysis. These chosen documents have been elaborated upon and are presented in Table 2, Table 3 and Table 4. Each of table provides a comprehensive summary of the selected documents, organized according to their respective clusters.
Reference | Description |
[27] | Presents a new approach for a universal robotic construction simulation by integrating ROS and BIM in an open simulator environment. Robot-based construction is viewed as one of the potential future solutions for achieving efficient construction. |
[28] | This article identifies the key functions representing the essential capabilities of BIM-AR platforms. It Identifies six key functions as the foundation for an evaluation framework that can assist practitioners in assessing the application area's requirements. |
[20] | Explores integration of RiC with other technologies such as building information modeling and deep learning. Identified the latest research topics and trends concerning RiC. Providing in-depth insights into the future direction of RiC |
[29] | Explores the potential of combining BIM and robotics for practical implementations in the AEC industries and the challenges it is facing. Mixed analysis (quantitative and qualitative) approach. |
[30] | Develops a software platform for data retrieval and analysis from BIM models and utilizing it efficiently during various stages of construction process. A framework is proposed to integrate BIM technology into automated construction through concrete 3D printing and was applied in a case study. |
[31] | Develops an integrated BIM-based indoor robot initialization system. Experiments in various environments using automatic initialization approach. |
[12] | Introduces a novel BIM methodology to facilitate the intricate geometry design and digital fabrication of modular housing units. |
[30] | Presents a software platform designed for data retrieval and analysis from BIM, with a focus on its efficient utilization across multiple stages of the construction process. |
[32] | An articulated robotic arm facilitates an efficient communication link between BIM-designed elements and additive concrete manufacturing. |
[33] | The study introduces a knowledge-driven approach to enable robotic agents to inspect autonomous facilities. |
[34] | Presents a novel BIM simulation-based method for assessing the automated assembly of wooden frames. The proposed approach offers a fresh perspective for professionals, providing a means to analyze building designs concerning the potential use of robotics for construction purposes. |
[35] | Introduces a method based on DRL for automated assembly planning within robot-based prefabricated construction. It involves the development of a re-configurable simulator for assembly planning, using BIM and open game engine. |
[36] | Involves a comprehensive examination of the information that can be directly extracted from the BIM and the additional data that needs to be integrated into the model to enable robots to execute specific tasks at the construction site. |
[37] | Designed a SLAM method to enhance the accuracy of positioning for the alignment of BIMs in AR presentations. |
[38] | Introduces a BIM-based LCA process designed to compare the carbon footprint of two design model options. The assessment considers various material choices, including the utilization of virgin materials as well as recycled and reused materials. |
[39] | Develops a construction operation simulation system by leveraging BIM technology and artificial intelligence. It also involves an analysis of simulation technology pertaining to the intelligent movement of construction operation mechanisms and the overall construction operations. |
[40] | Seeks to enhance the integration of BIM within the context of 3DCP processes. It comprehensively examines the technological compatibility and synergy between these two systems. |
[41] | Introduces a novel concept centered around the development of a systematic workflow for deriving a modular robotic system from a BIM model. |
[42] | Expands the scope of BIM to encompass the integration of robot task planning, facilitating the generation of intricate motion plans to execute construction tasks. |
[43] | Explores the potential of digital building platforms grounded in BIM to enhance OHS throughout the entire lifecycle of a building, including design, construction, operation, and deconstruction phases. |
[44] | This research examines the potential for incorporating contemporary digital technologies into modular construction methods to advance the goals of sustainable construction. The analysis specifically focuses on modular construction materials and their implications in achieving sustainability objectives. |
[45] | Proposed a novel approach by integrating computer vision with BIM to monitor the assembly process in modular construction factories. The method used object segmentation, aligning with BIM, and employing image processing to identify component installation. |
[46] | Focused on a significant challenge in robotic construction related to dimensional lumber and construction materials, specifically addressing the impact of material imperfections and manufacturing inaccuracies on the built structure compared to its digital counterpart. |
[47] | Established a framework for disassembly models by identifying essential parameters and utilizing BIM as the primary platform and graphic interface. The study explored the required information for building disassembly models and proposed relevant parameters implemented using BIM in a case study. |
[48] | Addressed the challenges in large-scale construction projects using AM. It presented an automated monitoring and controlled material extrusion method to enhance the stability of extrusion-based concrete 3D printing. |
[49] | Addressed challenges in digital workflows within the AEC industry, focusing on DtP end-to-end data flow. The proposed DtP workflow utilized a BIM approach based on the IFC schema to holistically interface construction, production, and machine-relevant data, incorporating digital human models, human abilities, safety, and ergonomic criteria. |
[50] | Explored the integration of LiDAR sensor technology and 3D BIM models for long-term SLAM in indoor, GPS-denied environments. |
[51] | Explored the integration of generative design with BIM in the construction industry, focusing on its application in prefabricated construction. It aimed to contribute to researchers and designers' understanding of the field and address future requirements. |
[52] | Introduced a BIM-based target value design method that extends the concept of sustainable design to early stages. Using a case study, the proposed methodology demonstrates its potential by achieving a substantial reduction of 1,240 kBTU/sf/year in annual energy consumption compared to traditional sustainable design approaches. |
[53] | Explored an innovative approach to robot localization in built environments by utilizing digital representations of BIM in the AEC industry. It stablished the feasibility of BIM-based robot localization, with discussions on error factors, including deviations between as-planned BIM and as-built status. |
[54] | Underscored the significance of progress monitoring in construction projects and the need for efficient techniques to ensure accurate analysis for project control. The paper classified and evaluated various progress monitoring methods based on the technology employed, highlighting their respective advantages and limitations. |
[55] | Explored the integration of light construction equipment into BIM-supported digital civil engineering, filling a gap where such integration has been limited. It provided a conceptualization of the linking between BIM and light construction equipment. |
[56] | They propose using a building information robotic system that utilizes BIM and industry foundation classes to create interoperable data between BIM and the robotic operating system. Validation through construction site experiments demonstrated the system's effectiveness in achieving smarter, safer, and more precise robot navigation. |
[57] | Contributed to the foundation of real-time DT for robotic construction processes in mixed reality, advancing knowledge in construction management and human-robot collaboration in digital fabrication. |
[58] | Presented a BIM-integrated indoor path planning framework for unmanned ground robots. It integrates robot information into BIM to create a knowledge base. Simulation results validated the effectiveness of this framework in addressing indoor path planning challenges for unmanned ground robots. |
[59] | Introduced the ontology for BIM-based robotic navigation and inspection tasks as an integrated ontology to facilitate logic-based inspection. |
BIM: building information models; ROS: robotic operating system; AR: augmented reality; RiC: robotics in construction; AEC: architecture, engineering, and construction; GPS: global positioning system; DRL: deep reinforcement learning; OHS: occupational health and safety; SLAM: simultaneous localization and mapping; DT: digital twin.
Reference | Description |
[60] | Endeavors to create a robust and dependable assessment methodology suitable for industrial applications, specifically designed to evaluate the sustainability of building construction projects that contemplate the utilization of CAR technologies. |
[61] | Offers a comparative analysis of the automation's effects on various urban areas in the United States. It suggests that smaller cities are likely to experience more pronounced adjustments, including worker displacement and shifts in job roles. |
[62] | Presents a practical, fully automated gantry-robot system designed for the efficient production of customized reinforcement cages. This system offers several advantages, including increased efficiency, productivity, sustainability, improved labor safety, and reduced environmental impact. |
[63] | Delves into the impact of emerging technologies, including BIM, GIS, VR, RFID, AI, ML, eye-tracking, serious games, and wearable devices, on the safety performance within the construction industry. |
[64] | Identifies and assesses the critical strategies for promoting the adoption of automation techniques within the context of the Nigerian construction industry. |
[65] | It highlights the importance of automation in the construction industry, particularly focusing on the relevance of emerging technologies such as IoT, automation, RFID, BIM, AR, and VR. |
[66] | Presents the outcomes of a Delphi study conducted to evaluate the expected level of technological knowledge that graduates should possess upon entering the industry. |
[67] | Employs the AHP technique to examine IR-4.0 technologies and their potential to address health and safety challenges within Malaysia's construction industry. |
[23] | Provides an extensive review of automation technology utilization in structural prefabrication and construction. It covers recent advancements, identifies challenges, and outlines future trends in this field. |
[68] | Introduces an efficient method for transferring pertinent data from a Building Information Model onto the construction site, directly enhancing the construction process by augmenting the site with design information. |
[69] | Introduces a framework for the automated evolution of robot morphology, eliminating the need for human intervention to adapt the robot to various HVAC and ceiling designs. |
[70] | Develops an anthropomorphic robot arm designed to mimic the movements and actions of a human arm. The integration of AI enhances the capabilities of these robotic arms, enabling them to make intelligent decisions and adapt to a wide range of tasks and functions. |
[71] | This research performs a narrative review to assess the current state-of-the-art in the use of robotic systems within the construction and manufacturing industries. |
[72] | Identifies significant initiatives that have harnessed intelligent tools to empower robots in elevating productivity, enhancing quality, and augmenting safety on construction sites. |
[73] | Presents a novel human gaze-aware hand gesture recognition framework for human-robot interaction in intelligent construction. The results show that this framework effectively facilitates one-to-many collaborations between humans and robots in construction tasks. |
[74] | Presents an automated inspection approach involving the development of an inspection robot and a dedicated management system. The inspection robot, featuring artificial intelligence sensors, a manipulator, and a ground vehicle, autonomously explores tunnels and employs stereo vision technology to assess damage on concrete surfaces. |
[75] | Describes the development of AcTEA-bot, a lightweight and replicable robotic system. AcTEA-bot is designed to autonomously identify and pinpoint thermal leaks within ceiling environments as it navigates. |
[76] | Presents a thorough review of the latest advancements in deep learning-based methods for semantic segmentation in construction applications. It is the first comprehensive survey in this domain, aligning these methods with the unique challenges faced by the construction industry. |
[77] | Investigates the principal technical, economic, legal, privacy, and resource barriers hindering the adoption of RPA in the context of tall building safety management. |
[78] | Introduces a robotic inspection system that leverages vision-based positioning and combines NDE measurements with pose information to identify and map subsurface defects. |
[79] | Examines the historical progression of robotics in construction and architecture and underscores the current shift from pure automation to more collaborative and adaptive processes. |
[80] | Introduces a novel HRI construction approach. It offers a new perspective within the realm of robotic construction research by merging the benefits of manual construction techniques with automated robotic construction methods. |
[81] | Presents a method for assessing the equilibrium status of masonry structures under construction, particularly those constructed using robotic technologies. |
[82] | Explored the use of ChatGPT in converting building code requirements into computer codes, comparing its performance with cutting-edge semantic rule-based approaches. The study claimed that ChatGPT has the potential to accelerate the implementation and scalability of automated building code compliance checking systems. |
[83] | Proposed a novel robot control methodology that directly utilizes fabrication information modeling data for controlling an AM system, specifically tailored to the needs of extrusion-based concrete 3D printing. |
[84] | Aimed to gather dynamic data on working process parameters to discern bulldozer operations, evaluate statistical characteristics of disturbances, and validate mathematical models for bulldozer processes. It proposed a method for digitizing experimental data rooted in the theory of random processes and digital signal processing. |
[85] | Introduced a real-time evaluator system to reduce costs and enhance automation by decreasing installation time, minimizing rework, and improving energy performance. The study claimed that the system holds potential for generating commands for autonomous crane operation or single-task construction robots. |
[86] | Aiming to introduce the lean AI method, this study developed through ongoing longitudinal studies analyzing AI implementations in the AEC industry, the method compels practitioners to articulate what AI should, can, and will solve early in the planning process. |
[4] | Provided an overview of the history of construction robotics and introduced the concept of robot-oriented design. It then delved into case studies from research projects and entrepreneurial ventures, showcasing the authors' involvement in advancing concrete construction. |
[87] | Explored the challenges, experiences, and lessons learned in the ongoing transformation of a masonry build system, traditionally reliant on manual labor, into a robot-automated build system. |
[88] | Introduced an automated process for on-site material management in the construction industry. The process involved selecting materials for staging based on the detection of construction progress from site images. Subsequently, a robot delivers the materials to their respective workface locations. |
[89] | Addressed the challenge of achieving zero energy consumption in existing buildings by proposing a solution that involved adding a new insulating envelope with renewable energy sources using prefabricated modules, automation, and robotics. |
[90] | Introduced a methodology for the comparative assessment of construction processes and the selection of an optimal solution based on automation implementation. The methodology combined case-based reasoning and compositional modeling to quantitatively evaluate construction processes. |
[91] | Discussed the challenges of robotic automation in construction sites, emphasizing the importance of human-robot collaboration for complex tasks. The approach facilitated intuitive programming of new tasks by allowing human demonstration through haptic interfaces. |
[92] | Introduced a novel method for AR-informed human-machine collaboration in timber prefabrication. The approach emphasized craftsman-controlled instructive interaction between a robotic fabrication setup and a human co-worker, aiming to enhance flexibility and robustness in automated workflows. |
[93] | Addressed the challenge of automatic compliance checking in the construction industry by converting regulatory documents and contract specifications from natural language to a machine-readable format. |
[94] | Discussed the challenges in reinforced concrete RC construction and emphasized the potential benefits of automation in enhancing worker safety, quality control, and overall efficiency. It presented a digital process for fabricating truss-like beams using 3D-printed clay molds, serving as formwork for the beam's cavities. |
[95] | Introduced a modular manufacturing platform with industrial robots, providing flexibility, reconfigurability, and transportability. The proposed system, comprising multiple modular platforms, can be adjusted to meet project requirements and set up on-site at local timber construction companies. |
[96] | Introduced an innovative approach to expanding the geometrical freedom of shotcrete 3D printing. Through quantitative investigations and identification of suitable parameter settings, the research enabled deterministic short-term printing gaps without nozzle blockage. |
BIM: building information models; CAR: construction automation and robotics; AR: augmented reality; GIS: geographic information system; RFID: radio frequency identification; VR: virtual reality; GPS: global positioning system; CAR: construction automation and robotics; HVAC: heating ventilation and air conditioning; SLAM: simultaneous localization and mapping; AI: artificial intelligence; AEC: architecture, engineering, and construction; RPA: robotic process automation; HRI: human-robot interaction; NDE: non-destructive evaluation; AHP: analytical hierarchy process; AM: additive manufacturing; ML: machine learning; IoT-IBIM: IoT-based Improved Building Information Modeling; RC-to-XR: reality capture to extended reality.
Reference | Description |
[97] | Presents a robotic system that combines perception sensors and advanced algorithms. Its purpose is to assist construction supervisors in remotely identifying construction materials, detecting component installations and defects, and generating detailed reports that include information on the status and location of these elements. |
[98] | Introduces an innovative grid-topological map and establishes a precise and efficient indoor pathfinding approach rooted in BIM. The map is designed by amalgamating the strengths of both grid-based mapping and topological mapping techniques. |
[99] | Underscores ongoing research endeavors aimed at integrating BIM into contour crafting construction methods. |
[100] | Conducts a comprehensive review of construction health and safety management technologies to uncover emerging research trends in the field. |
[101] | Introduces an automated quality verification system for modular BIM. The system comprises three main components: modular BIM object guidelines, a pre-checking module, and an automated code checking module. |
[102] | Presents an innovative method for remotely monitoring and evaluating the correct usage of PPE. This approach involves the integration of pressure sensors and positioning technologies. |
[103] | Presents a comprehensive framework for generating 4D animations of multi-mobile crane lift processes. By interacting with a central database, the approach can interface with a detailed BIM model or autonomously generate simplified CAD models, including lifting objects and obstacles. |
[104] | Introduces a BIM-centered system for automating the assembly of building components. The system is designed to align with sustainability principles within the construction industry and considers various factors to promote eco-friendly practices and maintain a sustainable environment. |
[105] | Suggests a framework based on BIM for the automated assessment of machine capabilities in the context of manufacturing construction-related products. |
[106] | Introduces an innovative computational method for identifying and reconstructing concrete defects using geotagged aerial images. The approach incorporates a bundle registration algorithm to align a set of aerial photos with a BIM. |
[107] | Seeks to pinpoint the crucial domains in which BIM can enhance and facilitate the clash management process throughout the entire lifecycle of a project. |
[108] | Investigate the relationship between LCA and BSA within the context of BIM in Portugal. An LCA for a specific Portuguese case study was conducted and simultaneously evaluating a set of sustainability criteria derived from SBTool throughout the assessment process. |
[109] | Introduces a semi-automated solution for capturing building exterior performance standards (BEPS) data for existing buildings. This solution can be integrated with other technologies like BIM or city GML and can be applied across an entire portfolio of buildings. |
[110] | Outlines the integration of BIM and BVBS using open BIM standards. This integration facilitates the seamless coordination of computerized design and the automation of steel reinforcement prefabrication processes. |
[111] | Introduces a semi-automated methodology for acquiring essential parameters needed to generate as-built BIM models for steel structures with intricate connections, leveraging data from terrestrial laser scanning. |
[112] | Emphasizes the integration of advanced technologies, including BIM, to propose principles and specific processes for a green intelligent building automation system. It also outlines the system's planning and design, rooted in practical engineering applications. |
[113] | Suggests an IoT-based Improved Building Information Modeling (IoT-IBIM) approach to address the challenges associated with building information modeling in contemporary project management. |
[114] | Establishes a comprehensive framework for automated construction progress monitoring, utilizing a DT approach. The framework spans from RC-to-XR, providing a versatile solution for construction monitoring. |
[115] | Introduces an automated approach rooted in BIM to minimize waste in roof sheathing material. The method enables proactive design and planning for the installation of roof sheathing in prefabricated buildings, reducing material waste. |
[116] | Presents a systematic approach, utilizing BIM, for 3D geometric modeling and automated generative design. The aim is to optimize both the carbon footprint and construction costs associated with offsite construction methods. |
[117] | Introduces an automated framework based on BIM for the real-time assessment and enhancement of sustainability in infrastructure projects. |
[118] | Delves into the pivotal role of blockchain technology in managing information related to actions, responsible parties, and timestamps. This, in turn, establishes a robust foundation for resolving potential legal conflicts. |
[119] | Provides an extensive review of design automation in civil engineering, with a specific focus on assessing building energy efficiency. It covers background information, practical applications, advantages, challenges, and suggests ways to enhance automation using AI. |
[65] | Underscores the importance of automation within the construction industry, especially in light of emerging technologies such as the IoT, automation, RFID, BIM, AR, VR. |
[120] | Delved into the definition and distinction between DT and BIM in the context of AEC industry and facilities management. It advocated for using DT to predict behavior and enhance construction management, operations, and maintenance practices. |
[121] | Addressed the slow implementation of robotic systems in the construction industry, which is characterized by low productivity and limited digitalization. It identified barriers to the introduction of robots, combining insights from existing literature with empirical findings from the German construction industry. |
[122] | Introduced a framework called Fabrication Information Modeling to enhance the planning efficiency of AM in the construction industry. The framework integrated AM seamlessly into the existing BIM methodology, allowing for efficient planning and enabling an end-to-end digital chain from project conception to production. |
[123] | This paper proposed a Blockchain-Encryption Integrated framework to enhance the security of BIM data when utilizing blockchain in collaborative projects. |
[124] | Introduced the BIM Interoperability Framework, a cloud-based platform designed to address interoperability challenges in the construction industry. The framework aimed to enable secure collaboration among legacy systems and modern applications within construction projects. |
[125] | Proposed a solution for BIM-based variant retrieval in the early design phases of building projects using case based reasoning and pattern matching. It aimed to enhance the efficiency of the design process, enable learning from past projects, and streamline the selection of appropriate design variants. |
[126] | Developed a methodology for evaluating project performance improvement through the appropriate automation of construction processes. The approach involved a quantitative evaluation using a compositional modeling-driven case-based reasoning methodology. |
[127] | Addressed the challenges of coordinating embeds in construction and explores different inspection processes, including traditional 2D paper inspection, AR + BIM inspection, and AR + BIM inspection with interactive queues. |
[128] | Presented the development of an on-site construction progress monitoring and presentation system that uses AR to overlay the virtual BIM onto the real-time construction site scene. |
[129] | Addressed the gaps in BIM by applying the concept of Productization and Product Structure. It aimed to enhance consistency in information flow within fragmented construction projects. |
[130] | The paper introduces an information quality assessment framework for BIM processes, emphasizing the importance of addressing both information completeness and correctness issues. |
[131] | Explored the challenges and strategies for implementing high-level autonomous robotic systems for on-site construction. It discussed technologies and algorithms used in construction robots and draws insights from robotic applications in other industries. |
[132] | Explored the potential of natural language processing and BIM to automate construction schedule management. It highlighted the challenges in manual scheduling and emphasizes the benefits of leveraging technologies for improved planning and scheduling. |
BIM: building information models; BEPS: building exterior performance standards; AR: augmented reality; VR: virtual reality; CAR: construction automation and robotics; RFID: radio frequency identification; AI: artificial intelligence; AEC: architecture, engineering, and construction; LCA: life cycle assessment; BSA: building sustainability assessment; AHP: analytical hierarchy process; AM: additive manufacturing; PPE: personal protective equipment; DT: digital twins.
2.4 Robotic in construction research dimensions and themes
In this research paper, the proposed framework for RiC involves categorizing the gathered research papers into various research dimensions. These dimensions are further subdivided into themes and sub-themes that define the specific research areas explored within RiC. Through an analysis of Figure 6, Figure 7, Figure 8 and Figure 9, three primary dimensions have been identified: Technology, Automation, and Sustainability, as summarized in Table 5. The Technology dimension includes studies in robotic construction that investigate the utilization of key technologies, including IoT[132], AR[37,133], VR[133,135], ML[136,138], DL[86,136,139-141], BIM[47,53,122,142], 3D printing[94,143-145], and sensor technologies[97], all of which play pivotal roles in the Robotic Construction Industry. In the Automation dimension, topics such as prefabricated construction[35], robotic arms[70], and concrete drilling[40] are thoroughly discussed, reflecting the automation aspects within the field. Lastly, the Sustainability dimension encompasses examinations of life cycle[25] characteristics and sustainable building design[104], particularly within the context of RiC field.
Dimensions | Frequent subjects | References |
Technology | VR-AR | [37,133-135] |
AI | [7,70,119] | |
IoT | [65,81,113,132] | |
DL | [35,86,136,137-141] | |
Sensor technology | [97,102] | |
BIM-3D printing | [47,53,94,142-145] | |
Automation | Prefabricated construction | [51,115,146-148] |
Robotic arms | [32,115] | |
Concrete drilling | [30] | |
Sustainability | Life cycle characteristics | [149-151] |
Sustainable building design | [104,116,152,153] |
BIM: building information models; RiC: robotics in construction; AR: augmented reality; VR: virtual reality; AI: artificial intelligence; DL: digital twins.
A list of ten themes has been identified as an appropriate classification system for the examined studies to refine these dimensions further. The proposed classification framework for RiC studies is outlined in Table 6. This framework includes associated references for each theme and a more detailed categorization of studies based on their specific research focus. The ten themes used for classifying RiC studies are as follows: automation and robotics in construction, BIM, sustainable construction, smart and resilient infrastructure, robotic material handling, data analytics and decision support, human-computer interaction, regulatory and ethical considerations, digital twins and simulation, and safety and risk management.
Themes | Sub-themes | References |
Automation and Robotics in Construction | Robotic Machinery | [105,154-156] |
Automation Technologies | [60,64,77,157,158] | |
Human-Robot Collaboration | [73,80,159,160] | |
BIM | BIM Integration | [40,42,110] |
BIM for Design and Analysis | [30,39,44] | |
Sustainable Construction | Energy Efficiency | [109,119,161-163] |
Life Cycle Assessment | [38,108,149-151] | |
Waste Reduction | [115,117] | |
Smart and Resilient Infrastructure | Smart Cities | [113,114,163,164] |
Resilience Infrastructure | [25,74] | |
Robotic Material Handling | Material Transportation | [38,88,115,165] |
3D Printing | [30,40,96,166,167] | |
Data Analytics and Decision Support | Big Data Analytics | [36,109] |
Decision Making | [105] | |
Human-Computer Interaction | User Interfaces | [22] |
Training and Skill Development | [66] | |
Regulatory and Ethical Considerations | Regulatory Frameworks | [71,80] |
Ethical Issues | [77,118] | |
Digital Twins and Simulation | Digital Twin Technology | [114,168-170] |
Simulation and Visualization | [27,39] | |
Safety and Risk Management | Safety | [23,77,100] |
Risk Assessment | [63,100] |
BIM: building information models; RiC: robotics in construction.
2.5 Classification of different robot types in construction
Construction robots can be sorted into different types based on their applications and uses. The following is a classification of some common types of robots that are used in the construction industry:
• Autonomous construction vehicles: such as dozers, excavators, load-carriers, and haul trucks. Those robots are usually created to perform various tasks such as drilling, leveling, material and object transportation, and site preparation. These robots can operate and navigate within construction sites autonomously[171].
• Automated guided vehicles (AGVs): They are used for handling and transporting materials on construction sites. AGVs autonomously follow predefined paths, where they can transfer materials between the areas of the work site[172].
• Unmanned aerial vehicles (UAVs): Drones are one of the most important and widespread examples of UAVs. They are utilized for surveying, mapping, monitoring, and inspecting construction sites. UAVs significance lies in providing real-time data, enhancing safety, and improving efficiency[173].
• Robotic arms: Robotic arms are flexible mechanical appendages that offer versatility through programmable functionalities, enabling them to undertake a multitude of tasks. In construction, they are used for tasks such as bricklaying, welding, concrete spraying, demolition, and assembly. These robots enhance precision, accelerate project timelines, and minimize labor-intensive efforts[174].
• Cable-driven parallel robots (CDPRs): These systems typically feature multiple cables connected to motors or winches at fixed points in the environment, enabling precise control over the position and orientation of the end-effector (the device used for tasks) within a defined workspace. CDPRs find application across various fields, including construction for tasks like assembly and material handling. Their capability to cover extensive workspaces with minimal structural support proves advantageous in scenarios where traditional robotic systems face impracticality or space constraints[175].
• Soft robots: They are crafted from pliable materials like silicone. In contrast to conventional rigid counterparts, soft robots boast enhanced versatility and the ability to replicate natural movements. They are usually used for applications such as inspection, maintenance, and manipulation in constrained spaces[176].
• Teleoperated robots: Teleoperated robots are controlled remotely by operators from a safe distance and are used for tasks that require human judgment and ingenuity. They can perform tasks such as remote inspection, manipulation, and operation of equipment in hazardous environments, thereby enhancing safety and productivity on construction sites[177].
• Construction 3D printers: 3D printing in construction involves the sequential layering of materials using computer-controlled processes to fabricate three-dimensional shapes. These printers typically utilize additive manufacturing techniques to construct building components. This technology proves invaluable for both on-site construction of new structures and off-site manufacturing of components, which are then assembled later, enabling the construction of complex shapes and structures[178].
• Exoskeletons: Exoskeletons are wearable machines suited with motorized joints that provide lift support for construction workers. They reduce strain, fatigue, and injury making it easier for workers to perform tasks involving heavy lifting or repetitive motions[179].
• AI-enabled construction robots: Equipped with cameras to monitor ongoing tasks, these robots leverage AI algorithms for decision-making, planning, and coordinating construction activities. Additionally, they optimize workflows, enhance resource allocation, and adeptly respond to changing site conditions[180].
• Demolition robots: Within the construction industry, demolition robots play a pivotal role in demolition and evacuation operations. Their significance lies in their ability to transport heavy materials from ground to elevated floors. Specialized robots for controlled demolition tasks, such as dismantling structures, breaking concrete, and clearing debris, ensure precision and safety in hazardous demolition environments[181].
Notably, this classification does not include other types of robots more related to the robot's capabilities than their types, such as AI-enabled robots. These robots are usually equipped with cameras to monitor ongoing tasks, they also leverage AI algorithms for decision-making, planning, and coordinating construction activities. Additionally, they optimize workflows, enhance resource allocation, and adeptly respond to changing site conditions[180].
3. Results and Discussion
In this section, the research provides answers to the questions that were initially posed at the outset, drawing upon the comprehensive literature review conducted.
In the context of research dimensions within the field of RiC, the study identifies three primary focal points: technology, automation, and environmental. A noteworthy observation is that research articles often concentrate on one or two of these dimensions, sometimes neglecting the holistic perspective. Consequently, the majority of documents tend to emphasize the technical and technological aspects of RiC, with comparatively less attention to its environmental implications. It's worth noting that this imbalance is less prevalent in review papers. Table 5 presents common subjects that frequently appear in the literature for each of these dimensions.
This research's primary objective was to refine further the dimensions previously identified in RiC research. This refinement was achieved by categorizing them into a set of themes and interrelated classes of research themes. Utilizing state-of-the-art technologies within RiC is one of the most rapidly expanding research themes in RiC, encompassing a substantial number of papers. These papers predominantly delve into various aspects of technology implementation in RiC, encompassing operations management of automation, robotic machinery, automation technologies, and issues related to human-robot collaboration. Following closely in terms of research activity are two additional highly dynamic themes: BIM and smart infrastructure. Within these themes, research efforts are notably concentrated on areas such as BIM integration, BIM applications for design and analysis, user interface developments, smart cities, and the resilience of infrastructure. It is noteworthy that several other themes, including sustainable construction, data analytics and decision support, robotic material handling, human-computer interaction, digital twins and simulation, and safety and risk management, predominantly feature papers that primarily address the technological aspects of RiC.
Three pivotal dimensions come to light within the automation and robotics in construction theme. The first dimension, robotic machinery, revolves around the development and utilization of specialized robotic equipment tailored for various construction tasks. Researchers aim to enhance the capabilities and efficiency of these machines, advancing the construction industry. The second dimension, automation technologies, delves into the application of cutting-edge automation technologies, including drones and autonomous vehicles, to streamline and optimize construction processes. This dimension propels research toward the seamless integration of these technologies into construction workflows. The third dimension, human-robot collaboration, addresses the crucial challenge of ensuring safe and productive collaboration between robots and human workers on construction sites. Research efforts within this realm focus on establishing protocols and mechanisms for harmonious coexistence and cooperation, contributing to a more productive and secure working environment.
BIM unfolds as another integral theme, encompassing two primary sub-themes: BIM integration emerges as the first dimension, emphasizing the seamless merging of BIM and robotics to augment project planning and management. Researchers in this domain strive to create a cohesive integration that enhances project efficiency and effectiveness. BIM for design and analysis forms the second dimension, focusing on harnessing BIM's potential for design optimization and structural analysis in construction projects. This dimension empowers researchers to leverage BIM for improved project outcomes.
Sustainable construction emerges as a theme enriched with various sub-themes. Energy Efficiency, the first dimension, concentrates research efforts on enhancing sustainability through robotic methods designed to optimize energy usage. Innovations in this realm seek to bolster energy performance and ecological sustainability. Eco-friendly materials, the second dimension, explores the integration of environmentally friendly construction materials into robotic processes, advancing construction practices aligned with eco-conscious principles. Waste reduction, the third dimension, employs robotics and automation to minimize construction waste and reduce the environmental impact. Researchers in this realm implement strategies to align construction activities with sustainability objectives.
The theme of safety and risk management unfolds with two integral dimensions. Safety robotics, the first dimension, centers on the development of robots and systems designed to enhance safety on construction sites. This dimension strives to reduce the risk of accidents and injuries, contributing to improved safety standards. Risk assessment, the second dimension, harnesses robotics and AI for assessing and mitigating risks associated with construction projects. Researchers delve into this domain to enhance risk management practices within the construction industry.
Smart and resilient infrastructure emerges as another critical theme, comprising two significant dimensions. Smart cities, the first dimension, explores the applications of RiC in advancing smart city infrastructure. Researchers within this dimension emphasize the pivotal role of robotics in creating technologically advanced urban environments. Resilience, the second dimension, focuses on strategies and methodologies aimed at fortifying infrastructure resilience through the integration of robotics. Research within this realm contributes to the development of infrastructure capable of withstanding and recovering from adverse events and challenges.
The theme of digital twins and simulation unfolds with two substantial dimensions. Digital twin technology, the first dimension, involves the creation of digital replicas of physical construction projects for analysis and optimization. This dimension facilitates comprehensive analysis, optimizing project performance and efficiency. Simulation and visualization, the second dimension, employs simulation and visualization tools in construction project planning and monitoring. These tools aid in visualizing processes and making informed decisions during construction.
The robotic material handling theme encompasses two dimensions. Material transportation, the first dimension, focuses on the automation of material handling and transportation processes on construction sites, enhancing efficiency and productivity. 3D printing, the second dimension, explores advancements in 3D printing technologies tailored specifically for construction purposes, revolutionizing the precision and automation of building component fabrication.
Data analytics and decision support constitute a theme with two critical dimensions. Big data analytics, the first dimension, emphasizes the analysis of construction data for informed decision-making, underscoring the importance of harnessing big data in construction projects. Predictive analytics, the second dimension, shifts the focus toward using AI and ML techniques to predict project outcomes and optimize resource allocation, enhancing planning and decision support in construction.
The theme of human-computer interaction includes two vital dimensions. User interfaces, the first dimension, centers on the design and development of intuitive user interfaces, enhancing the control and monitoring of construction robots and ensuring efficient human-robot interaction. Training and skill development, the second dimension, underscores research efforts aimed at designing training programs and skill development initiatives for workers operating robotic systems within the construction industry, ultimately improving their competence and proficiency.
Finally, the theme of regulatory and ethical considerations comprises two essential dimensions. Regulatory frameworks, the first dimension, delves into the development and adherence to regulations governing robotic construction activities, including the establishment of guidelines and standards to ensure the safe and compliant usage of robotics in construction. Ethical issues, the second dimension, explores the ethical implications associated with the adoption and deployment of robotic technologies in the construction industry, taking into account potential societal impacts and ethical considerations that arise. These themes and sub-themes encompass the multidisciplinary aspects of robotics in construction and can serve as a basis for research and innovation in the field.
Research in the field of RiC is continually evolving, but several gaps and challenges persist. Identifying these gaps can help guide future research efforts and innovation in the field. A comprehensive perspective that encompasses all three dimensions of RiC is imperative for a thorough comprehension of this field. Several studies persist in concentrating solely on one or two of the recognized dimensions, and some even fall short in providing a coherent roadmap for the integration of technology in RiC that ensures the incorporation and consideration of the remaining two dimensions. This analysis of RiC studies highlights a potential blind spot in the domain of ICT services and information systems, which could impede cohesion within robotic systems.
In the domain of RiC, numerous critical research gaps have emerged, necessitating further investigation and attention. Firstly, despite the potential for RiC to contribute to sustainable construction practices, a significant research gap exists in quantifying and optimizing the environmental benefits associated with its implementation. This gap hinders the ability to fully realize the potential environmental advantages of RiC technologies. Secondly, there is a notable absence of long-term studies assessing the performance and durability of structures constructed using robotic technologies. This raises concerns about the longevity and reliability of such projects, highlighting the need for more extensive research in this area to ensure the long-term viability of RiC applications. Thirdly, the integration of robotics into conventional construction workflows without causing disruption remains a challenge that requires further exploration. Many construction sites still heavily rely on traditional methods, and the seamless incorporation of robotic systems into existing processes presents logistical and operational challenges that need to be addressed.
Furthermore, the absence of standardized practices and specific regulations tailored to RiC poses a hindrance to its widespread adoption. The development of industry standards and regulatory guidelines is essential to address this gap and facilitate the smooth implementation of RiC technologies across different construction projects. The safe interaction between robots and human workers is another crucial concern, and current research efforts have been insufficient in developing advanced safety protocols and effective collaborative mechanisms to address this challenge. Ensuring the safety of workers in environments where robots operate is paramount and requires further research and development efforts. Moreover, the affordability and accessibility of robotic solutions, particularly for smaller construction firms, are issues that have not been adequately addressed. Research focusing on cost-effective and scalable robotic systems is imperative to democratize access to RiC technologies and promote their widespread adoption across the construction industry.
Given the substantial data generated by RiC, there is a pressing need for research aimed at developing efficient data management, analytics, and visualization tools to harness the full potential of this data. As robotic technologies become increasingly prevalent in construction, the lack of adequate training programs and skill development for workers to effectively operate and interact with robotic systems poses a significant challenge. Ethical considerations related to job displacement, privacy concerns, and the broader societal impacts of RiC demand thorough examination to ensure responsible and ethical deployment of these technologies.
It's important to acknowledge that while RiC holds promise for revolutionizing the construction industry, there are several inherent limitations that need to be considered. For instance, the compatibility issues between traditional construction techniques and robotic systems can restrain the adoption of RiC technologies. Additionally, the lack of standardized protocols and interoperability among different robotic systems further complicates their integration into existing construction workflows. Manufacturers use different systems; it becomes more challenging for interoperability and impedes the development of a cohesive wide industry procedure. Many robotic systems lack the required adaptability to navigate the different circumstances found on construction sites. Robotic technologies are still not responding effectively to changes in the environment, unexpected conditions. Thus, the varied requirements of different projects are considered a complicated challenge for robotic technologies.
Identifying and addressing these research gaps is crucial to advancing the field of robotics in construction and realizing its potential to transform the construction industry. Researchers, industry professionals, and policymakers should collaborate to tackle these challenges effectively.
Finally, in terms of recommendations for future studies, it's imperative to address the identified gaps and limitations in RiC applications. Research efforts should focus on developing efficient data management, analytics, and visualization tools to harness the full potential of RiC data for improved decision-making and project management. Furthermore, there is a need for comprehensive research aimed at developing advanced safety protocols, industry standards, and regulatory guidelines tailored to RiC. Additionally, research focusing on the development of user-friendly interfaces and ergonomic designs for construction robots can enhance their usability and acceptance among workers, ultimately contributing to the successful integration of RiC into construction practices.
4. Conclusion and Future Direction
This paper conducted content analysis on more than 180 journal articles to elucidate the primary dimensions and subjects within the domain of RiC. This review aimed to address the main dimensions of the RiC field that the majority of the relevant studies focused on during the last six years. Then it defined the themes and subthemes associated with these dimensions. Lastly, the main gaps in the current studies were listed and good future directions and research horizons of the RiC field were discussed. By introducing a classification framework and conducting extensive bibliometric and bibliographic analyses on papers published within the previous six years, various research trends within the domain of RiC were uncovered. The bibliometric analysis unveiled four primary keyword clusters that encapsulate the central themes of RiC research: "Robotic," "BIM," "automation," "environment," and "technology." In contrast, the bibliographic analysis shed light on key aspects of RiC, encompassing IT technologies, sustainable construction practices, smart infrastructure, smart cities, and robotic materials handling.
The insights provided in this study offer guidance for future research endeavors, helping to align RiC with a clearly defined set of dimensions, categories, and research classes. Acknowledging a limitation related to the interpretative nature of the proposed categorization framework, which may introduce subject bias, is essential. Furthermore, the framework presented in this work may not fully encompass integrated and holistic approaches, warranting further exploration in future research.
From the recommendations derived from the developed framework, it is anticipated that future research in this domain should prioritize areas such as sustainability, energy efficiency, and conducting life cycle assessments of RiC projects. Hence, it is imperative to further investigate the potential of RiC in constructing highly efficient buildings to discern its genuine impact on environmental sustainability. There is currently a dearth of long-term studies that assess the performance and longevity of structures constructed through robotic technologies. Additionally, an emerging direction for the future involves the establishment of industry standards and regulatory guidelines, as well as the exploration of cost-effective and scalable robotic systems. Construction sites face challenges in integrating robotic technologies due to compatibility issues with traditional methods and lack of standardized protocols. Diverse manufacturing systems hinder interoperability, and many robots struggle to adapt to different construction site conditions, affecting their effectiveness in responding to environmental changes and project-specific requirements. Lastly, collaborative research efforts involving experts from diverse fields, including robotics, construction, materials science, etc., will be crucial for effectively addressing the intricate challenges within RiC.
Authors contribution
Masri AA: Conceptualization, methodology, software, formal analysis, data curation, writing-original draft, writing-review and editing.
da Costa BBF, Vasco D: Conceptualization, validation, investigation, data curation, writing-review and editing, supervision.
Boer D: Conceptualization, methodology, validation, formal analysis, investigation, data curation, writing-review and editing, supervision.
Haddad AN: Conceptualization, methodology, validation, formal analysis, investigation, data curation, writing-review and editing, visualization, supervision.
Najjar MK: Conceptualization, methodology, software, validation, formal analysis, investigation, data curation, writing-review and editing, visualization, supervision.
All authors have read and agreed to the published version of the manuscript.
Conflicts of interest
The authors declare no conflicts of interest.
Ethical approval
Not applicable.
Consent to participate
Not applicable.
Consent to publication
Not applicable.
Availability of data and materials
Not applicable.
Funding
This research was funded by CAPES Foundation (Coordination for the Improvement of Higher Education Personnel).
Copyright
© The Author(s) 2024.
References
-
1. Lundeen KM, Kamat VR, Menassa CC, McGee W. Scene understanding for adaptive manipulation in robotized construction work. Autom Constr. 2017;82:16-30.
[DOI] -
2. Bock T. Construction robotics. Auton Robots. 2007;22:201-209.
[DOI] -
3. Saidi KS, Bock T, Georgoulas C. Robotics in construction. In: Siciliano B, Khatib O, editors. Springer handbook of robotics. Cham: Springer; 2016. p. 1493-1520.
[DOI] -
4. Hu R, Pan W, Iturralde K, Linner T, Bock T. Construction automation and robotics for concrete construction: case studies on research, development, and innovations. In: 2023 Proceedings of the 40th ISARC; 2023 Jul 5-7; Chennai, India. Chennai: IAARC; 2023. p. 683-690.
[DOI] -
5. Baduge SK, Thilakarathna S, Perera JS, Arashpour M, Sharafi P, Teodosio B, et al. Artificial intelligence and smart vision for building and construction 4.0: Machine and deep learning methods and applications. Autom Constr. 2022;141:104440.
[DOI] -
6. Klarin A, Xiao Q. Automation in architecture, engineering and construction: a scientometric analysis and implications for management. Eng Constr Archit Manag. 2024;31(8):3308-3334.
[DOI] -
7. Pan Y, Zhang L. Roles of artificial intelligence in construction engineering and management: a critical review and future trends. Autom Constr. 2021;122(1):103517.
[DOI] -
8. Sánchez-Garrido AJ, Navarro IJ, García J, Yepes V. A systematic literature review on modern methods of construction in building: An integrated approach using machine learning. J Build Eng. 2023;73:106725.
[DOI] -
9. Anane W, Iordanova I, Ouellet-Plamondon C. Building information modeling (BIM) and robotic manufacturing technological interoperability in construction-a cyclic systematic literature review. Digital Manuf Technol. 2023;3(1):1-29.
[DOI] -
10. Gradeci K, Labonnote N. On the potential of integrating building information modelling (BIM) for the additive manufacturing (AM) of concrete structures. Constr Innov. 2020;20(3):321-343.
[DOI] -
11. Ding L, Jiang W, Zhou Y, Zhou C, Liu S. BIM-based task-level planning for robotic brick assembly through image-based 3D modeling. Adv Eng Inf. 2020;43:100993.
[DOI] -
12. He R, Li M, Gan VJL, Ma J. BIM-enabled computerized design and digital fabrication of industrialized buildings: a case study. J Cleaner Prod. 2021;278:123505.
[DOI] -
13. Ravichandar H, Polydoros AS, Chernova S, Billard A. Recent advances in robot learning from demonstration. Annu Rev Control Robot Auton Syst. 2020;3:297-330.
[DOI] -
14. Kumar R. An analysis on resource-Efficient approach for 3D printing in robotics. J Green Eng. 2020;10(9):6326-6333. Available from: https://www.scopus.com/inward/record.uri?eid=2-s2.0-85094178596&partnerID=40&md5=54193fd9b924f5323aef082b9bfdcf77
-
15. Egli P, Hutter M. A general approach for the automation of hydraulic excavator arms using reinforcement learning. IEEE Robot Autom Lett. 2022;7(2):5679-5686.
[DOI] -
16. Elghaish F, Matarneh S, Talebi S, Kagioglou M, Hosseini MR, Abrishami S. Toward digitalization in the construction industry with immersive and drones technologies: a critical literature review. Smart Sustain Built Environ. 2021;10(3):345-363.
[DOI] -
17. Zhao H, Hu S, Chen Q, Tao G, Dai J. A novel framework for automation technology Based on machine vision and robotics in electrical power inspection processing. Appl Bionics Biomech. 2022;2022:9158936.
[DOI] -
18. Ahonen A, de Koning M, Machado T, Ghabcheloo R, Sievi-Korte O. An exploratory study of software engineering in heavy-duty mobile machine automation. Robt Auton Syst. 2023;165(C):104424.
[DOI] -
19. Guray TS, Kismet B. VR and AR in construction management research: bibliometric and descriptive analyses. Smart Sustain Built Environ. 2023;12(3):635-659.
[DOI] -
20. Xiao B, Chen C, Yin X. Recent advancements of robotics in construction. Autom Constr. 2022;144:104591.
[DOI] -
21. Gharbia M, Chang-Richards A, Lu Y, Zhong RY, Li H. Robotic technologies for on-site building construction: A systematic review. J Build Eng. 2020;32:101584.
[DOI] -
22. Brissi SG, Chong OW, Debs L, Zhang J. A review on the interactions of robotic systems and lean principles in offsite construction. Eng Constr Archi Manage. 2022;29(1):383-406.
[DOI] -
23. Chea CP, Bai Y, Pan X, Arashpour M, Xie Y. An integrated review of automation and robotic technologies for structural prefabrication and construction. Transp Saf Environ. 2020;2(2):81-96.
[DOI] -
24. Pan Y, Zhang L. Roles of artificial intelligence in construction engineering and management: A critical review and future trends. Autom Constr. 2021;122:103517.
[DOI] -
25. Hoeft M, Pieper M, Eriksson K, Bargstädt HJ. Toward life cycle sustainability in infrastructure: the role of automation and robotics in PPP projects. Sustainability. 2021;13(7):3779.
[DOI] -
26. Scopus, Scopus Preview [Internet]. 2023. Available from: https://www.scopus.com
-
27. Zhu A, Pauwels P, De Vries B. Component-based robot prefabricated construction simulation using IFC-based building information models. Autom Constr. 2023;152:104899.
[DOI] -
28. Amin K, Mills G, Wilson D. Key functions in BIM-based AR platforms. Autom Constr. 2023;150:104816.
[DOI] -
29. Zhang J, Luo H, Xu J. Towards fully BIM-enabled building automation and robotics: A perspective of lifecycle information flow. Comput Ind. 2022;135:103570.
[DOI] -
30. Davtalab O, Kazemian A, Khoshnevis B. Perspectives on a BIM-integrated software platform for robotic construction through contour crafting. Autom Constr. 2018;89:13-23.
[DOI] -
31. Zhao X, Cheah CC. BIM-based indoor mobile robot initialization for construction automation using object detection. Autom in Constr. 2023;146:104647.
[DOI] -
32. Forcael E, Pérez J, Vásquez Á, García-Alvarado R, Orozco F, Sepúlveda J. Development of communication protocols between bim elements and 3D concrete printing. Appl Sci. 2021;11(16):7226.
[DOI] -
33. Chen J, Lu W, Fu Y, Dong Z. Automated facility inspection using robotics and BIM: A knowledge-driven approach. Adv Eng Inf. 2023;55:101838.
[DOI] -
34. Chong OW, Zhang J, Voyles RM, Min BC. BIM-based simulation of construction robotics in the assembly process of wood frames. Autom Constr. 2022;137:104194.
[DOI] -
35. Zhu A, Dai T, Xu G, Pauwels P, De Vries B, Fang M. Deep reinforcement learning for real-time assembly planning in robot-based prefabricated construction. IEEE Trans Autom Sci Eng. 2023;20(3):1515-1526.
[DOI] -
36. McClymonds A, Asadi S, Wagner A, Leicht RM. Information Exchange for Supporting BIM to Robotic Construction. In: Construction Research Congress 2022; 2022 Mar 9-12; Arlington, Virginia. Reston: American Society of Civil Engineers; 2022. p. 839-848.
[DOI] -
37. Hsieh CC, Chen HM, Wang SK. On-site Visual Construction Management System Based on the Integration of SLAM-based AR and BIM on a Handheld Device. KSCE J Civ Eng. 2023;27(11):4688-4707.
[DOI] -
38. Feng H, Chen Q, de Soto BG, Arashpour M. Using BIM and LCA to evaluate material circularity: Contributions to building design improvements. In: 2022 Proceedings of the 39th ISARC; 2022 Jul 13-15; Bogotá, Colombia. Colombia: IAARC; 2022. p. 9-16.
[DOI] -
39. Cai H. Building construction operation simulation based on BIM technology and intelligent robots. J Interconnect Netw. 2022;22(03):2145005.
[DOI] -
40. Anane W, Iordanova I, Ouellet-Plamondon C. The use of BIM for robotic 3D concrete printing. In: Walbridge S, Nik-Bakht M, Ng KTW, Shome M, Alam MS, Ashraf El, Damatty AE, Lovegrove G, editors. Proceedings of the Canadian Society of Civil Engineering Annual Conference 2021. Singapore: Springer; 2021. p. 325-336.
[DOI] -
41. Han S, Menzel K. Modular robotic system for the construction industry. In: Hjelseth E, Sujan SF, Scherer RJ, editors. ECPPM 2022-eWork and eBusiness in Architecture, Engineering and Construction 2022. London: CRC Press. 2023. p. 493-499.
[DOI] -
42. Kim S, Peavy M, Huang PC, Kim K. Development of BIM-integrated construction robot task planning and simulation system. Autom Constr. 2021;127:103720.
[DOI] -
43. Hoeft M, Trask C. Safety built right in: exploring the occupational health and safety potential of BIM-based platforms throughout the building lifecycle. Sustainability. 2022;14(10):6104.
[DOI] -
44. Smorzhenkov N, Ignatova E. Sustainability of construction based on digital and modular technologies. EDP Sciences. 2023;410:04006.
[DOI] -
45. Panahi R, Louis J, Podder A, Pless S, Swanson C, Jafari M. Automated progress monitoring in modular construction factories using computer vision and building information modeling. In: 40th International Symposium on Automation and Robotics in Construction, ISARC 2023; 2023 Jul 5-7; Chennai, India. Chennai: IAARC; 2023. p. 1-8.
[DOI] -
46. Ruan D, McGee W, Adel A. Reducing uncertainty in multi-robot construction through perception modelling and adaptive fabrication. In: 2023 Proceedings of the 40th ISARC; 2023 Jul 5-7; Chennai, India. Chennai: IAARC; 2023. p. 25-31.
[DOI] -
47. Sanchez B, Herthogs P, Stouffs R. Identifying key parameters for BIM-based disassembly planning. In: 2023 Proceedings of the 40th ISARC; 2023 Jul 5-7; Chennai, India. Chennai: IAARC; 2023. p. 32-39.
[DOI] -
48. Mohiuddin R, Cruz Policroniades M, Slepicka M, Borrmann A. Optical process control for extrusion-based additive manufacturing methods in construction. In: 2023 Proceedings of the 40th ISARC; 2023 Jul 5-7; Chennai, India. Chennai: IAARC; 2023. p. 94-101.
[DOI] -
49. Schmailzl M, Spitzhirn M, Eder F, Krüll G, Linner T, Obergrießer M, et al. Towards interfacing human centered design processes with the AEC industry by leveraging BIM-based planning methodologies. In: 2023 Proceedings of the 40th ISARC; 2023 Jul 5-7; Chennai, India. Chennai: IAARC; 2023. p. 325-332.
[DOI] -
50. Torres MAV, Braun A, Borrmann A. Bim-slam: Integrating bim models in multi-session slam for lifelong mapping using 3d lidar. In: 2023 Proceedings of the 40th ISARC; 2023 Jul 5-7; Chennai, India. Chennai: IAARC; 2023. p. 521-528.
[DOI] -
51. Rangasamy V, Yang JB. Generative design for prefabricated structures using BIM and IoT applications-approaches and requirements. In: 2023 Proceedings of the 40th ISARC; 2023 Jul 5-7; Chennai, India. Chennai: IAARC; 2023. p. 644-651.
[DOI] -
52. Saurav B, Diya K, Salonee R, Chen Q. Using BIM to facilitate generative target value design for energy efficient buildings. In: 2023 Proceedings of the 40th ISARC; 2023 Jul 5-7; Chennai, India. Chennai: IAARC; 2023. p. 667-674.
[DOI] -
53. Yin H, Liew JM, Lee WL, Ang MH. Yeoh JKW. Towards BIM-based robot localization: a real-world case study. In: 2022 Proceedings of the 39th ISARC; 2022 Jul 13-15; Bogotá, Colombia. Bogotá: IAARC; 2022. p. 71-77.
[DOI] -
54. Reja VK, Pradeep MS, Varghese K. A systematic classification and evaluation of automated progress monitoring technologies in construction. In: 2022 Proceedings of the 39th ISARC; 2022 Jul 13-15; Bogotá, Colombia. Bogotá: IAARC; 2022. p. 120-127.
[DOI] -
55. Schöberl M, Offinger S, Goldfuß T, Kessler S, Fottner J. BIM-integration of light construction equipment. In: 2022 Proceedings of the 39th ISARC; 2022 Jul 13-15; Bogotá, Colombia. Bogotá: IAARC; 2022. p. 183-190.
[DOI] -
56. Karimi S, Braga RG, Iordanova I, St-Onge D. Semantic optimal robot navigation using building information on construction sites. In: 2021 Proceedings of the 38th ISARC; 2021 Nov 2-4; Dubai, UAE. Dubai: IAARC; 2022. p. 57-64.
[DOI] -
57. Ravi KSD, Ng SM, Ibáñez JM, Hall DM. Real-time digital twin of on-site robotic construction processes in mixed reality. In: 2021 Proceedings of the 38th ISARC; 2021 Nov 2-4; Dubai, UAE. Dubai: IAARC; 2022. p. 451-458.
[DOI] -
58. Chen Z, Chen K, Cheng JCP. A BIM-integrated indoor path planning framework for unmanned ground robot. In: 2021 Proceedings of the 38th ISARC; 2021 Nov 2-4; Dubai, UAE. Dubai: IAARC; 2022. p. 776-783.
[DOI] -
59. Bahreini F, Hammad A. Towards an ontology for BIM-Based robotic navigation and inspection tasks. In: 2021 Proceedings of the 38th ISARC; 2021 Nov 2-4; Dubai, UAE. Dubai: IAARC; 2022. p. 1025-1032.
[DOI] -
60. Pan M, Linner T, Pan W, Cheng H, Bock T. A framework of indicators for assessing construction automation and robotics in the sustainability context. J Clean Prod. 2018;182:82-95.
[DOI] -
61. Frank MR, Sun L, Cebrian M, Youn H, Rahwan I. Small cities face greater impact from automation. J R Soc Interface. 2018;15(139):20170946.
[DOI] -
62. Momeni M, Relefors J, Khatry A, Pettersson L, Papadopoulos AV, Nolte T. Automated fabrication of reinforcement cages using a robotized production cell. Autom Constr. 2022;133:103990.
[DOI] -
63. Dobrucali E, Demirkesen S, Sadikoglu E, Zhang C, Damci E. Investigating the impact of emerging technologies on construction safety performance. Eng Constr Archit Manage. 2024;31(3):1322-1347.
[DOI] -
64. Oke AE, Aliu J, Fadamiro P, Akanni P, Jamir Singhr PS, Shaharudin Samsurijan M. Unpacking the strategies to promote the implementation of automation techniques in the construction industry. Constr Innov. 2023;25(2):381-399.
[DOI] -
65. Srivastava A, Jawaid S, Singh R, Gehlot A, Akram SV, Priyadarshi N, et al. Imperative role of technology intervention and implementation for automation in the construction industry. Adv Civ Eng. 2022;2022(2):1-19.
[DOI] -
66. Tayeh R, Issa RRA. Developing construction information systems courses based on collaboration between industry and academia. J Archit Eng. 2021;27(3):04021016.
[DOI] -
67. Musarat MA, Alaloul WS, Irfan M, Sreenivasan P, Rabbani MBA. Health and safety improvement through Industrial Revolution 4.0: Malaysian construction industry case. Sustainability. 2022;15(1):201.
[DOI] -
68. Degani A, Li WB, Sacks R, Ma L. An automated system for projection of interior construction layouts. IEEE Trans Autom Sci Eng. 2019;16(4):1825-1835.
[DOI] -
69. Duan K, Suen CWK, Zou Z. Robot morphology evolution for automated HVAC system inspections using graph heuristic search and reinforcement learning. Autom Constr. 2023;153:104956.
[DOI] -
70. Devi SN, Prabha KL, Ezhilazhagan C, Sivakumar SA, Tamilarasu R, Kumar SS, et al. Integration of Artificial Intelligence into Operations and Monitoring of 3R Manipulator. Interl J Intell Syst Appl Eng. 2023;11(9s):591-597. Available form: https://ijisae.org/index.php/IJISAE/article/view/3199
-
71. Tehrani BM, BuHamdan S, Alwisy A. Robotics in assembly-based industrialized construction: A narrative review and a look forward. Int J Intell Rob Appl. 2023;7(3):556-574.
[DOI] -
72. Attalla A, Attalla O, Moussa A, Shafique D. Construction robotics: review of intelligent features. Int J Intell Rob Appl. 2023;7(3):535-555.
[DOI] -
73. Wang X, Veeramani D, Zhu Z. Gaze-aware hand gesture recognition for intelligent construction. Eng Appl Artif Intell. 2023;123:106179.
[DOI] -
74. Shim S, Lee SW, Cho GC, Kim J, Kang SM. Remote robotic system for 3D measurement of concrete damage in tunnel with ground vehicle and manipulator. Comput Aided Civ Infrastruct Eng. 2023;38(15):2180-2201.
[DOI] -
75. Cai W, Huang L, Zou Z. Actively-exploring thermography-enabled autonomous robotic system for detecting and registering HVAC thermal leaks. Autom Constr. 2023;152:104901.
[DOI] -
76. Rauch L, Braml T. Semantic point cloud segmentation with deep-learning-based approaches for the construction industry: A survey. Appl Sci. 2023;13(16):9146.
[DOI] -
77. Waqar A, Othman I, Falqi II, Almujibah HR, Alshehri AM, Alsulamy SH, et al. Assessment of barriers to robotics process automation (RPA) implementation in safety management of tall buildings. Buildings. 2023;13(7):1663.
[DOI] -
78. Hoxha E, Feng J, Sanakov D, Xiao J. Robotic inspection and subsurface defect mapping using impact-echo and ground penetrating radar. IEEE Robot Autom Lett. 2023;8(8):4943-4950.
[DOI] -
79. Parascho S. Construction robotics: From automation to collaboration. Annu Rev Control Rob Auton Syst. 2023;6:183-204.
[DOI] -
80. Leng Y, Shi X, Hiroatsu F. Automated construction for human-robot interaction in wooden buildings: Integrated robotic construction and digital design of iSMART wooden arches. J Field Rob. 2023;40(4):810-827.
[DOI] -
81. Paris V, Ruscica G, Olivieri C, Mirabella Roberti G. A systemic approach to simulate the construction process of self-supporting masonry structures. Sustainability. 2023;15(12):9596.
[DOI] -
82. Zhang J. How can ChatGPT help in automated building code compliance checking? In: 2023 Proceedings of the 40th ISARC; 2023 Jul 5-7; Chennai, India. Chennai: IAARC; 2023. p. 63-70.
[DOI] -
83. Slepicka M, Helou J, Borrmann A. Real-time data exchange (RTDE) robot control integration for Fabrication Information Modeling. In: 2023 Proceedings of the 40th ISARC; 2023 Jul 5-7; Chennai, India. Chennai: IAARC; 2023. p. 102-109.
[DOI] -
84. Bulgakov A, Bock T, Tokmakov G, Emelianov S. Bulldozer sensing technique for the purpose of automation for bulldozer's workflow. In: 2023 Proceedings of the 40th ISARC; 2023 Jul 5-7; Chennai, India. Chennai: IAARC; 2023. p. 180-185.
[DOI] -
85. Hun DE, Wang PL, Puente BPM, Hayes NW. Automated tracking of prefabricated components for a real-time evaluator to optimize and automate installation. In: 40th International Symposium on Automation and Robotics in Construction (ISARC); 2023 Jul 4-7; Chennai, India. Oak Ridge: Oak Ridge National Laboratory; 2023. p. 192-199.
[DOI] -
86. Agrawal A, Singh V, Fischer M. LeanAI: A method for AEC practitioners to effectively plan AI implementations. In: 2023 Proceedings of the 40th ISARC; 2023 Jul 5-7; Chennai, India. Chennai: IAARC; 2023. p. 652-659.
[DOI] -
87. Klöckner M, Haage M, Eriksson H, Malm H, Nilsson K, Robertsson A, et al. Insights into automation of construction process using parallel-kinematic manipulators. In: 2022 Proceedings of the 39th ISARC; 2022 Jul 13-15; Bogotá, Colombia. Colombia: IAARC; 2022. p. 25-32.
[DOI] -
88. Stephans T, McClymonds A, Leicht R, Wagner A. Automated material selection based on detected construction progress. In: 2022 Proceedings of the 39th ISARC; 2022 Jul 13-15; Bogotá, Colombia. Colombia: IAARC; 2022. p. 406-413.
[DOI] -
89. Iturralde K, Zimmermann P, Santos RGB, Das S, Shen W, Malik M, et al. Solution Kits for automated and robotic façade upgrading. In: 2022 Proceedings of the 39th ISARC; 2022 Jul 13-15; Bogotá, Colombia. Colombia: IAARC; 2022. p. 414-421.
[DOI] -
90. Krishnamoorthi S, Raphael B. A case-based reasoning technique for evaluating performance improvement in automated construction projects. In: 2022 Proceedings of the 39th ISARC; 2022 Jul 13-15; Bogotá, Colombia. Colombia: IAARC; 2022. p. 590-596.
[DOI] -
91. Brosque C, Herrero EG, Chen Y, Joshi R, Khatib O, Fischer M. Collaborativewelding and joint sealing robots with haptic feedback. In: 2021 Proceedings of the 38th ISARC; 2021 Nov 2-4; Dubai, UAE. Dubai: IAARC; 2022. p. 1-8.
[DOI] -
92. Amtsberg F, Yang X, Skoury L, Wagner HG, Menges A. iHRC: an AR-based interface for intuitive, interactive and coordinated task sharing between humans and robots in building construction. In: 2021 Proceedings of the 38th ISARC; 2021 Nov 2-4; Dubai, UAE. Dubai: IAARC; 2022. p. 25-32.
[DOI] -
93. Schönfelder P, König M. Deep learning-based entity recognition in construction regulatory documents. In: 2021 Proceedings of the 38th ISARC; 2021 Nov 2-4; Dubai, UAE. Dubai: IAARC; 2022. p. 387-394.
[DOI] -
94. Maitenaz S, Charrier M, Mesnil R, Onfroy P, Metge N, Feraille A, et al. Fabrication of a truss-like beam casted with 3D printed clay moulds. In: 2021 Proceedings of the 38th ISARC; 2021 Nov 2-4; Dubai, UAE. Dubai: IAARC; 2022. p. 712-716.
[DOI] -
95. Kaiser B, Littfinski D, Verl A. Automatic generation of digital twin models for simulation of reconfigurable robotic fabrication systems for timber prefabrication. In: 2021 Proceedings of the 38th ISARC; 2021 Nov 2-4; Dubai, UAE. Dubai: IAARC; 2022. p. 717-724.
[DOI] -
96. Lachmayer L, Dörrie R, Kloft H, Raatz A. Automated shotcrete 3D printing-Printing interruption for extended component complexity. In: 2021 Proceedings of the 38th ISARC; 2021 Nov 2-4; Dubai, UAE. Dubai: IAARC; 2022. p. 725-732.
[DOI] -
97. Ilyas M, Khaw HY, Selvaraj NM, Jin Y, Zhao X, Cheah CC. Robot-assisted object detection for construction automation: Data and information-driven approach. IEEE/ASME Trans Mechatronics. 2021;26(6):2845-2856.
[DOI] -
98. Zhou X, Xie Q, Guo M, Zhao J, Wang J. Accurate and efficient indoor pathfinding based on building information modeling data. IEEE Trans Ind Inf. 2020;16(12):7459-7468.
[DOI] -
99. Akhnoukh AK. Advantages of contour crafting in construction applications. Recent Pat Eng. 2021;15(3):294-300.
[DOI] -
100. Akinlolu M, Haupt TC, Edwards DJ, Simpeh E. A bibliometric review of the status and emerging research trends in construction safety management technologies. Int J Constr Manage. 2022;22(14):2699-2711.
[DOI] -
101. Sun H, Kim I. Automated checking system for modular BIM objects. J Civ Eng Manage. 2022;28(7):554-563.
[DOI] -
102. Dong S, Li H, Yin Q. Building information modeling in combination with real time location systems and sensors for safety performance enhancement. Saf Sci. 2018;102:226-237.
[DOI] -
103. Shahnavaz F, Taghaddos H, Najafabadi RS, Hermann U. Multi crane lift simulation using building information modeling. Autom Constr. 2020;118:103305.
[DOI] -
104. Wang W. Automatic system design of assembly building components for sustainable building projects based on BIM technology. Math Probl Eng. 2022;2022:1-11.
[DOI] -
105. An S, Martinez P, Al-Hussein M, Ahmad R. BIM-based decision support system for automated manufacturability check of wood frame assemblies. Autom Constr. 2020;111:103065.
[DOI] -
106. Chen J, Lu W, Lou J. Automatic concrete defect detection and reconstruction by aligning aerial images onto semantic-rich building information model. Comput Aided Civ Infrastruct Eng. 2023;38(8):1079-1098.
[DOI] -
107. Fawaz M, Ibrahim R, Rashidi A, Ghafar MA. From modelling to management of project delivery: review on clash management process with BIM in malaysia. Malays Constr Res J. 2021;35(3):57-70. Available from: https://www.scopus.com/inward/record.uri?eid=2-s2.0-85129794549&partnerID=40&md5=66829ed445d9fca2bee1896ba62eeb3b
-
108. Carvalho JP, Alecrim I, Bragança L, Mateus R. Integrating BIM-based LCA and building sustainability assessment. Sustainability. 2020;12(18):7468.
[DOI] -
109. O'Donnell J, Truong-Hong L, Boyle N, Corry E, Cao J, Laefer DF. LiDAR point-cloud mapping of building façades for building energy performance simulation. Autom Constr. 2019;107:102905.
[DOI] -
110. Liu Y, Li M, Wong BCL, Chan CM, Cheng JCP, Gan VJL. BIM-BVBS integration with openBIM standards for automatic prefabrication of steel reinforcement. Autom Constr. 2021;125:103654.
[DOI] -
111. Yang L, Cheng JCP, Wang Q. Semi-automated generation of parametric BIM for steel structures based on terrestrial laser scanning data. Autom Const. 2020;112:103037.
[DOI] -
112. Wang H, Shen Y, Niu Y, Zheng Y. Design and research of building automation system and its system planning for green intelligent building. J Environ Prot Ecol. 2019;20(2):832-841. Available from: https://www.scopus.com/inward/record.uri?eid=2-s2.0-85082101654&partnerID=40&md5=c70100763dacbe0cefe2bff895ba45aa
-
113. Lian M, Liu X. Significance of building information modeling in modern project management for sustainable smart city applications. J Interconnect Netw. 2022;22:2141007.
[DOI] -
114. Alizadehsalehi S, Yitmen I. Digital twin-based progress monitoring management model through reality capture to extended reality technologies (DRX). Smart Sustain Built Environ. 2021;12(1):200-236.
[DOI] -
115. Liu H, Sydora C, Altaf MS, Han SH, Al-Hussein M. Towards sustainable construction: BIM-enabled design and planning of roof sheathing installation for prefabricated buildings. J Cleaner Prod. 2019;235:1189-1201.
[DOI] -
116. Gan VJL. BIM-based building geometric modeling and automatic generative design for sustainable offsite construction. J Constr Eng Manage. 2022;148(10):04022111.
[DOI] -
117. Laali A, Nourzad SHH, Faghihi V. Optimizing sustainability of infrastructure projects through the integration of building information modeling and envision rating system at the design stage. Sustain Cities Soc. 2022;84:104013.
[DOI] -
118. Sayed B, Oral HV. A review on blockchain operations in construction management. J Sustain Constr Mater Technol. 2023;8(2):146-152.
[DOI] -
119. Khairulzaman HA, Usman F. Automation in civil engineering design in assessing building energy efficiency. Int J Eng Technol. 2018;7:722-727.
[DOI] -
120. Correa FR. BIM, twin and between: conceptual engineering approach to formalize digital twins in construction. In: 2022 Proceedings of the 39th ISARC; 2022 Jul 13-15; Bogotá, Colombia. Colombia: IAARC; 2022. p. 475-482.
[DOI] -
121. Jäkel JI, Rahnama S, Klemt-Albert K. Construction robotics excellence model: a framework to overcome existing barriers for the implementation of robotics in the construction industry. In: 2022 Proceedings of the 39th ISARC; 2022 Jul 13-15; Bogotá, Colombia. Colombia: IAARC; 2022. p. 605-613.
[DOI] -
122. Slepicka M, Vilgertshofer S, Borrmann A. Fabrication information modeling: closing the gap between building information modeling and digital fabrication. In: 2021 Proceedings of the 38th ISARC; 2021 Nov 2-4; Dubai, UAE. Dubai: IAARC; 2021. p. 9-16.
[DOI] -
123. Tao X, Das M, Liu Y, Yiu PWK, Chen K, Cheng JCP. A conceptual framework for secure BIM-based design: using blockchain and asymmetric encryption. In: 2021 Proceedings of the 38th ISARC; 2021 Nov 2-4; Dubai, UAE. Dubai: IAARC; 2021. p. 81-87.
[DOI] -
124. Bountouni N, Lampathaki F, Kousouris S, Tsitsanis A, Vafeiadis G, Vergeti D. The BIMERR interoperability framework: towards BIM enabled interoperability in the construction Sector. In: 2021 Proceedings of the 38th ISARC; 2021 Nov 2-4; Dubai, UAE. Dubai: IAARC; 2021. p. 94-101.
[DOI] -
125. Napps D, Pawlowski D, König M. BIM-based variant retrieval of building designs using case-based reasoning and pattern matching. In: 2021 Proceedings of the 38th ISARC; 2021 Nov 2-4; Dubai, UAE. Dubai: IAARC; 2021. p. 435-442.
[DOI] -
126. Krishnamoorthi S, Raphael B. A case based reasoning approach for selecting appropriate construction automation method. In: 2021 Proceedings of the 38th ISARC; 2021 Nov 2-4; Dubai, UAE. Dubai: IAARC; 2021. p. 880-885.
[DOI] -
127. Kim J, Olsen D. From BIM to inspection: a comparative analysis of assistive embedment inspection. In: 2021 Proceedings of the 38th ISARC; 2021 Nov 2-4; Dubai, UAE. Dubai: IAARC; 2021. p. 909-915.
[DOI] -
128. Wang SK, Chen HM. A construction progress on-site monitoring and presentation system based on the integration of augmented reality and BIM. In: 2020 Proceedings of the 37th ISARC; 2020 Oct 27-28; Kitakyushu, Japan. Kitakyushu: IAARC; 2020. p. 147-154.
[DOI] -
129. Mansoori S, Haapasalo H, Härkönen J. On construction-specific product structure design and development: the BIM enhancement approach. In: 2020 Proceedings of the 37th ISARC; 2020 Oct 27-28; Kitakyushu, Japan. Kitakyushu: IAARC; 2020. p. 177-184.
[DOI] -
130. Chen LJ, Yeoh JKW. An information quality assessment framework for developing building information models. In: 2020 Proceedings of the 37th ISARC; 2020 Oct 27-28; Kitakyushu, Japan. Kitakyushu: IAARC; 2020. p. 207-214.
[DOI] -
131. Xu X, de Soto BG. On-site autonomous construction robots: A review of research areas, technologies, and suggestions for advancement. In: 2019 Proceedings of the 36th ISARC; 2020 Oct 27-28; Banff, Canada. Banff: IAARC; 2020. p. 385-392.
[DOI] -
132. Natephra W, Motamedi A. Live data visualization of IoT sensors using augmented reality (AR) and BIM. In: 2019 Proceedings of the 36th ISARC; 2020 Oct 27-28; Banff, Canada. Banff: IAARC; 2019. p. 632-638.
[DOI] -
133. Park J, Chang S, Lee H, Cho Y. Inspection data exchange and visualization for building maintenance using AR-enabled BIM. In: 2022 Proceedings of the 39th ISARC; 2022 Jul 13-15; Bogotá, Colombia. Colombia: IAARC; 2022. p. 483-490.
[DOI] -
134. Eiris R, Gheisari M. iVisit-Collaborate: Online multiuser virtual site visits using 360-degree panoramas and virtual humans. In: 2022 Proceedings of the 39th ISARC; 2022 Jul 13-15; Bogotá, Colombia. Colombia: IAARC; 2022. p. 276-282.
[DOI] -
135. Dalence ARR, Vahdatikhaki F, Miller S, Dorée A. Integrating VR and simulation for enhanced planning of asphalt compaction. In: 2022 Proceedings of the 39th ISARC; 2022 Jul 13-15; Bogotá, Colombia. Colombia: IAARC; 2022. p. 55-62.
[DOI] -
136. Nevatia A, Saha S, Bhagavatula SB, Bugalia N. A pre-trained language model-based framework for deduplication of construction safety newspaper articles. In: 2023 Proceedings of the 40th ISARC; 2023 Jul 5-7; Chennai, India. IAARC Publications; 2023. p. 387-394.
[DOI] -
137. Shen Q, Vahdatikhaki F, Miller SR, Doree A. Coupling asphalt construction process quality into product quality using data-driven methods. In: 2023 Proceedings of the 40th ISARC; 2023 Jul 5-7; Chennai, India. Chennai: IAARC; 2023. p. 349-356.
[DOI] -
138. Kayhani N, McCabe B, Sankaran B. BIM-based construction quality assessment using Graph Neural Networks. In: 2023 Proceedings of the 40th ISARC; 2023 Jul 5-7; Chennai, India. Chennai: IAARC; 2023. p. 9-16.
[DOI] -
139. Nguyen HAD, Le TH, Ha QP, Azzi M. Deep learning for construction emission monitoring with low-cost sensor network. In: 2023 Proceedings of the 40th ISARC; 2023 Jul 5-7; Chennai, India. Chennai: IAARC; 2023. p. 450-457.
[DOI] -
140. Chen Y, Kim S, Ahn Y, Cho YK. A framework of reconstructing piping systems on classimbalanced 3D point cloud data from construction sites. In: 2023 Proceedings of the 40th ISARC; 2023 Jul 5-7; Chennai, India. Chennai: IAARC; 2023. p. 426-433.
[DOI] -
141. Kim J, Paik S, Lian Y, Kim J, Kim H. Transformation of point clouds to images for safety analysis of scaffold joints. In: 2023 Proceedings of the 40th ISARC; 2023 Jul 5-7; Chennai, India. Chennai: IAARC; 2023. p. 365-370.
[DOI] -
142. Esposito MB, Bosché F. Building information model pre-processing for automated geometric quality control. In: 2022 Proceedings of the 39th ISARC; 2022 Jul 13-15; Bogotá, Colombia. Colombia: IAARC; 2022. p. 17-24.
[DOI] -
143. Sati ASE, Mantha BRK, Dabous SA, de Soto BG. Classification of robotic 3D printers in the AEC industry. In: 2021 Proceedings of the 38th ISARC; 2021 Nov 2-4; Dubai, UAE. Dubai: IAARC; 2021. p. 924-931.
[DOI] -
144. Núñez C, Regalado M, Gago A. 3D printing: an opportunity for the sustainable development of building construction. In: 2023 Proceedings of the 40th ISARC; 2023 Jul 5-7; Chennai, India. Chennai: IAARC; 2023. p. 691-698.
[DOI] -
145. AlBalkhy W, Bing S, El-Babidi S, Lafhaj Z, Ducoulombier L. The analysis of lean wastes in construction 3D printing: A case study. In: 2023 Proceedings of the 40th ISARC; 2023 Jul 5-7; Chennai, India. Chennai: IAARC; 2023. p. 621-628.
[DOI] -
146. Dong M, Yang B, Liu B, Wang Z, Zhang B. Realizing, Twinning, and applying IFC-based 4D construction management information model of prefabricated buildings. In: 2021 Proceedings of the 38th ISARC; 2021 Nov 2-4; Dubai, UAE. Dubai: IAARC; 2021. p. 137-144.
[DOI] -
147. Rener A, Karatas A, Cole M. Innovative model for forecasting trailer usage for prefabricated exterior wall panels. In: 2022 Proceedings of the 39th ISARC; 2022 Jul 13-15; Bogotá, Colombia. Colombia: IAARC; 2022. p. 144-151.
[DOI] -
148. Iturralde K, Amburi S, Ravichandran S, Das S, Liu D, Bock T. Online modelling and prefab layout definition for building renovation. In: 2023 Proceedings of the 40th ISARC; 2023 Jul 5-7; Chennai, India. Chennai: IAARC; 2023. p. 605-608.
[DOI] -
149. Forth K, Abualdenien J, Borrmann A, Fellermann S, Schunicht C. Design optimization approach comparing multicriterial variants using BIM in early design stages. In: 2021 Proceedings of the 38th ISARC; 2021 Nov 2-4; Dubai, UAE. Dubai: IAARC; 2021. p. 235-242.
[DOI] -
150. Giorgadze IM, Vahdatikhaki F, Voordijk H. Conceptual modeling of lifecycle digital twin architecture for bridges: A data structure approach. In: 2022 Proceedings of the 39th ISARC; 2022 Jul 13-15; Bogotá, Colombia. Colombia: IAARC; 2022. p. 199-206.
[DOI] -
151. Ramprasad P, Dave B, Zilliacus M, Viranj P. Sustainability in Construction Projects: Setting and measuring impacts. In: 2023 Proceedings of the 40th ISARC; 2023 Jul 5-7; Chennai, India. Chennai: IAARC; 2023. p. 722-729.
[DOI] -
152. Hammad AWA, Wu P, Hammad M, Haddad A, Wang X. Mathematical optimisation of rail station location and route design in urban regions through minimising noise pollution. In: 2018 Proceedings of the 35th ISARC; 2018 Jul 20-25; Berlin, Germany. Berlin: IAARC; 2018. p. 1-6.
[DOI] -
153. Tan T, Papadonikolaki E, Mills G, Chen J, Zhang Z, Chen K. BIM-enabled sustainability assessment of design for manufacture and assembly. In: 2021 Proceedings of the 38th ISARC; 2021 Nov 2-4; Dubai, UAE. Dubai: IAARC; 2021. p. 849-856.
[DOI] -
154. Bock T, Aseev V, Bulgakov A. Overview of robotic shotcrete technologies with basalt reinforcement. In: 2023 Proceedings of the 40th ISARC; 2023 Jul 5-7; Chennai, India. Chennai: IAARC; 2023. p. 609-612.
[DOI] -
155. Bulgakov A, Otto J, Yu W, Rimshin V, Shleenko A. Structural organization of robotic building and mounting complexes. In: 2023 Proceedings of the 40th ISARC; 2023 Jul 5-7; Chennai, India. Chennai: IAARC; 2023. p. 208-213.
[DOI] -
156. Ambrosino M, Boucher F, Mengeot P, Garone E. Full-scale prototype for bricklaying activity. In: 2023 Proceedings of the 40th ISARC; 2023 Jul 5-7; Chennai, India. Chennai: IAARC; 2023. p. 79-85.
[DOI] -
157. Abanes JRY, Prieto SA, de Soto BG. Autonomous and continuous as-is 3D thermal mapping for construction environments. In: 2023 Proceedings of the 40th ISARC; 2023 Jul 5-7; Chennai, India. Chennai: IAARC; 2023. p. 16-19.
[DOI] -
158. Klöckner M, Haage M, Andersson R, Nilsson K, Robertsson A, Eriksson H. Bettan-Industrial robot and application for Finja Exakt build system. In: 2023 Proceedings of the 40th ISARC; 2023 Jul 5-7; Chennai, India. Chennai: IAARC; 2023. p. 86-93.
[DOI] -
159. Chae Y, Gupta S, Ham Y. Human-robot interaction in sloping jobsites (Demolition): Exploring impact of visual interfaces. In: 2023 Proceedings of the 40th ISARC; 2023 Jul 5-7; Chennai, India. Chennai: IAARC; 2023. p. 5-7.
[DOI] -
160. Garshasbi A, Wang J, Chen J, Ma J. Human-robot collaboration in the construction industry: A mini-review. In: ICRA 2023 Future of Construction Workshop Papers; 2023 Jun 2; London, UK. London: IAARC; 2023. p. 1-4.
[DOI] -
161. Zhang YY, Hu ZZ, Lin JR, Zhang JP. Data cleaning for prediction and its evaluation of building energy consumption. In: 2021 Proceedings of the 38th ISARC; 2021 Nov 2-4; Dubai, UAE. Dubai: IAARC; 2021. p. 427-434.
[DOI] -
162. Li H, Zhang J. IFC-based information extraction and analysis of HVAC objects to support building energy modeling. In: 2022 Proceedings of the 39th ISARC; 2022 Jul 13-15; Bogotá, Colombia. Colombia: IAARC; 2022. p. 159-166.
[DOI] -
163. Zhang H, Sun F, Feng J, Ling H, Zhou D, Cao G, et al. A stable, self-regulating, flexible, ITO-free electrochromic smart window for energy-efficient buildings. Cell Rep Phys Sci. 2022;3(12):101193.
[DOI] -
164. Ghosh A, Hafnaoui R, Mesloub A, Elkhayat K, Albaqawy G, Alnaim AA, et al. Active smart switchable glazing for smart city: A review. J Build Eng. 2024;84:108644.
[DOI] -
165. Dhanani N, Vignesh VP, Venkatachalam S. Demonstration of LiDAR on accurate surface damage measurement: a case of transportation infrastructure. In: ICRA 2023 Future of Construction Workshop Papers; 2023 Jun 2; London, UK. London: IAARC; 2023. p. 553-560.
[DOI] -
166. Xu X, Wang R, Cao Q, Feng C. Towards 3D perception and closed-Loop control for 3D construction printing. In: 2020 Proceedings of the 37th ISARC; 2020 Oct 27-28; Kitakyushu, Japan. Kitakyushu: IAARC; 2020. p. 1576-1583.
[DOI] -
167. Senthilnathan S, Raphael B. Quality monitoring of concrete 3D Printed elements using computer vision-based texture extraction technique. In: 2023 Proceedings of the 40th ISARC; 2023 Jul 5-7; Chennai, India. Chennai: IAARC; 2023. p. 474-481.
[DOI] -
168. Yamasaki F. Digital twin technology: Utilizing robots and deep learning. In: 2020 Proceedings of the 37th ISARC; 2020 Oct 27-28; Kitakyushu, Japan. Kitakyushu: IAARC; 2020. p. 1523-1527.
[DOI] -
169. Chellappa V, Chauhan JS. Digital twin approach for the ergonomic evaluation of vertical formwork operations in construction. In: 2023 Proceedings of the 40th ISARC; 2023 Jul 5-7; Chennai, India. Chennai: IAARC; 2023. p. 302-308.
[DOI] -
170. Paul A, Pulani S, Maheswari JU. Digital twin framework for worker safety using RFID technology. In: 2023 Proceedings of the 40th ISARC; 2023 Jul 5-7; Chennai, India. Chennai: IAARC; 2023. p. 254-261.
[DOI] -
171. Morikawa H, Otsuki T. Study on the level concept of autonomous construction in mechanized construction. In: 2020 Proceedings of the 37th ISARC; 2020 Oct 27-28; Kitakyushu, Japan. Kitakyushu: IAARC; 2020. p. 1114-1121.
[DOI] -
172. Zhang J, Yang X, Wang W, Guan J, Ding L. Automated guided vehicles and autonomous mobile robots for recognition and tracking in civil engineering. Autom Constr. 2023;146:104699.
[DOI] -
173. Freimuth H, König M. Planning and executing construction inspections with unmanned aerial vehicles. Autom Constr. 2018;96:540-553.
[DOI] -
174. Zeman Z, Mihola M, Suder J, Boleslavsky A. Automation of partial tasks in the design of robotic ARMS. MM Sci J. 2022;1:5513-5521.
[DOI] -
175. Gueners D, Chanal H, Bouzgarrou BC. Design and implementation of a cable-driven parallel robot for additive manufacturing applications. Mechatronics. 2022;86:102874.
[DOI] -
176. Atia MGB, Mohammad A, Gameros A, Axinte D, Wright I. Modelling of modular soft robots: From a single to multiple building blocks. Rob Auton Syst. 2024;174:104622.
[DOI] -
177. Koh KH, Farhan M, Yeung KPC, Tang DCH, Lau MPY, Cheung PK, et al. Teleoperated service robotic system for on-site surface rust removal and protection of high-rise exterior gas pipes. Autom Constr. 2021;125:103609.
[DOI] -
178. Rehman AU, Kim IG, Kim JH. Towards full automation in 3D concrete printing construction: Development of an automated and inline sensor-printer integrated instrument for in-situ assessment of structural build-up and quality of concrete. Dev Built Environ. 2024;17:100344.
[DOI] -
179. Cai M, Ji Z, Li Q, Luo X. Safety evaluation of human–robot collaboration for industrial exoskeleton. Saf Sci. 2023;164:106142.
[DOI] -
180. Arkouli Z, Kokotinis G, Michalos G, Dimitropoulos N, Makris S. AI-enhanced cooperating robots for reconfigurable manufacturing of large parts. IFAC-Pap Online. 2021;54(1):617-622.
[DOI] -
181. Mu Z, Liu L, Jia L, Zhang L, Ding N, Wang C. Intelligent demolition robot: Structural statics, collision detection, and dynamic control. Autom Constr. 2022;142:104490.
[DOI]
Copyright
© The Author(s) 2024. This is an Open Access article licensed under a Creative Commons Attribution 4.0 International License (https://creativecommons.org/licenses/by/4.0/), which permits unrestricted use, sharing, adaptation, distribution and reproduction in any medium or format, for any purpose, even commercially, as long as you give appropriate credit to the original author(s) and the source, provide a link to the Creative Commons license, and indicate if changes were made.
Publisher’s Note
Share And Cite