- 1 Department of Chemistry, Lahore Garrison University, DHA Phase VI, Lahore 54792, Pakistan.
- 2 Department of Chemistry, Division of Science and Technology, University of Education, Lahore 44770, Pakistan.
Abstract
Integration of inorganic fillers with polymer matrices opens up exciting possibilities for creating high-performance functional materials. Metal/metal oxides/silsesquioxanes as nanofillers are gaining attention for enhancing polymer matrices. Understanding the dispersion and interaction of these nanofillers is crucial for modifying properties in technological applications. Homogeneously dispersed fillers boost physiochemical and biological properties, like mechanical strength and biocompatibility. These functional materials are employed in tissue engineering and other healthcare applications, maintaining their characteristics even in challenging conditions. In the biomedical field, these fillers show promise in developing stimuli-responsive systems, drug delivery, gene vectors, and biological imaging.
Keywords
1. Introduction
In the past decades, nanomaterial with one dimension less than 100 nm attracted much attention due to novel and promising applications in different area of interest. This is because combination of polymer and nanofillers afford a number of possibilities for the design of new materials with technological importance. Macroscopic performance of the new materials from synergy of polymer and nanofillers strongly depend on the later dispersion in the former. Different approaches are used to overcome nanofillers aggregation either by functionalization or by selection of a decent processing method (e.g. ultrasonication, mechanical blending, rapid precipitation, to name a few)[1]. To make nanofillers capable for different applications, control on the geometrical distribution is necessary, which require compatibility with polymer matrix, external fields, templates or polymer modification. The dispersion of these nanofillers takes place both in water (hydrosols) or organic solvents (organosols), depending on the conditions and capping agent in the surrounding[2]. To avoid irreversible growth and agglomeration of the metal oxide nanoparticles we should protect its reactive surface-by-surface ligands. Thus, the fabrication of nanofillers with proper dispersion and controlled structure is indispensable and dependent on the selection of the ligands. A variety of motifs can be used for this purpose which includes surfactants, oligomers, polymers etc., and different combinations and motifs can be achieved through non-covalent interactions (e.g. electrostatic forces, acid-base proton transfer, hydrogen bonding and other van der Waal forces)[3]. Metal oxide particles display interesting application potential which stem from their exciting size dependent optoelectronic and physicochemical properties[4,5]. Recently intensive technological and scientific efforts are being carried out to uniformly disperse metal oxide nanoparticles into organic polymer matrix and thereby fabricating organic-inorganic (O-I) hybrid materials[6,7]. For this purpose different strategies are used, but one of the successful strategy is the colloidal dispersion and encapsulation of nanoparticles with silica shells or polyhedral oligomeric silsesquioxane (POSS) cubes[8]. POSS, with hydrophobic moieties that can be linked to hydrophilic surfaces either of metal/metal oxides or polymers, for the development of ordered structures like self-assembly in bulk, solution, and thin films such as core shell micelles, vesicle, cylinders, microphase separated nanostructures[9-15]. These moieties have the additional advantages of controlling distance between the core particles in the assemblies, biocompatible, permeability, ease of functionalization, bioconjugation, oxidative stability, flame retardency, and non-covalent modifiers[8,15-21]. Coalescence of properties of inorganic filler and polymer matrix provides fascinating and economic approach for tailoring the desired high performance materials[22,23]. A variety of polymer-metal oxide nanocomposites that offer attractive thermal, mechanical, electrical, and optical properties has been extensively studied[24]. Polyhedral oligomeric silsesquioxane (POSS) are three-dimensional, molecular level organic-inorganic architectures consisting of alternate Si-O bonds forming the cage structures with R forming the periphery. The peripheral moieties can be exploited for mono and multi-functional POSS preparation. Based on the compatibility, structure and reactivity of the peripheral moieties, POSS can be incorporated homogeneously into polymer matrices.
Properties of POSS related composite materials are dependent on well dispersed and uniform incorporation of POSS particles into polymer matrices of interest. Usually two approaches are used to afford nanocomposites with well-dispersed POSS particles i.e. (i) chemical cross-linking and (ii) physical blending[15,25-27]. Very little is known about metal oxide/POSS nanocomposites. Some researchers have studied metal/POSS nanocomposites for antimicrobial and catalytic applications[3,8,28-32]. Different technological properties of the developed nanocomposites are dependent on the coating, of the nanoparticles with suitable coating agent. Covalent attachments of nanoparticles and polymer backbones are found to enhance the thermal and mechanical properties[1,18,33-36]. Some researchers have studied the optical, electronic, and magnetic properties to be dependent on the inter-particles spacing and ordered assemblies[37,38]. To cut short due to stable, ease of isolation, molecular and variously functionalization chemistry of POSS, its composites with metal are preferred over metal/polymer composites[39-41]. Keeping these in view different properties of metal/metal oxides/silsesquioxane based polymer composites are reviewed here.
2. Metal/Metal Oxide/Silsesquioxane Nanoparticles Chemistry
The two key properties of nanoparticles i.e. large number of unsaturated surface atoms and quantum size effects compared to bulk materials make its chemistry interesting for different technological applications[42]. Due to their high surface energy, aggregation is the major challenge in achieving these desired applications. Different researchers have tried to overcome these challenges as reviewed follows:
2.1 Stable dispersion of nanoparticles in aqueous and non-aqueous media
Scientific implementation, utilization of nanoparticles into different commercial products ranging from catalysts, food safety, clinical diagnosis, environmental monitoring, UV-blocking coating, sunscreens, composite materials require robust and cost-effective homogenous dispersion, compatibility with different organic polymer matrices, and surface functionalization in aqueous and non-aqueous media[43,44]. To make these particles effective as Nano fillers, they are stabilized in the carrier liquid and prevented from air oxidation, agglomeration by their surface modification with different additives[45-49]. NP-mediated applications requires surface functionalization that not only affects their solubility, dispersion, stability, and compatibility but also provide the cationic, anionic and neutral functional groups or charges that are exploited for intermolecular, van der Waals, ligand exchange and other interactions. Nanoparticles are mostly used in Nano fluids e.g. iron nanoparticles used as Ferro fluids due to their greater saturation magnetization. Iron nanoparticles can be stabilized in the carrier liquid to avoid agglomeration and air oxidation by coating with a surfactant or a polymer[50-52]. D. Maity, D.C. Agrawal have studied iron oxide nanoparticles in aqueous as well as non-aqueous media[53]. Qiao, Ruirui, et al. grafted iron oxide nanoparticles with bromomaleimide and PEG brush polymers in non-aqueous medium for biomedical applications[54]. Hola, Katerina, et al. have highlighted different covalent and non-covalent strategies for the functionalization and stabilization of iron oxide nanoparticles[55]. They have discussed three aspects of functionalization by incorporating common functional groups i.e. chemical modification of amine groups, bioactive substances, and carboxyl groups for enzyme immobilization on iron oxide nanoparticles surface. Lee, S.H., Nishi, H., & Tatsuma, T. have tuned the plasmon resonance of molybdenum oxide nanoparticles in non-aqueous media for visible range electronic harvesting materials[56]. Patil, R.M., et al. have functionalized iron oxide nanoparticles with oleic acid to reduce their agglomeration followed by treatment with betaine-HCl to increase their hydrophilicity and stability for hyperthermia application[57]. In another study collagen fibers are cross-linked with oleic acid functionalized iron oxide nanoparticles and were employed for oil removal application in non-aqueous media[58]. Kharisov, Boris I., et al. have reviewed the recent trends in solubilization and stabilization of iron nanoparticles in aqueous and non-aqueous media[59]. Some researchers have adopted dodecylamine protected silver nanoparticles using tin (II) acetate as reducing agent and SnO2 nanoparticles for electronic ink formulation in non-aqueous medium[60,61]. Kharissova, Oxana V., Boris I. Kharisov, and Edgar Gerardo de Casas Ortiz. have reviewed dispersion of carbon nanotubes in aqueous and non-aqueous media with great emphasizes on physical techniques and using surface agents[62]. Kaushik, Madhu, and Audrey Moores. reviewed nano-cellulose as support for functional metal nanoparticles for catalytic applications[63]. Wang, Shunzhi, et al. have reported the progress in metal organic frameworks as functional nanomaterials for sensing, membranes applications based on porosity, and diverse chemical functionalities[64]. Park, Jong-Won, and Jennifer S. Shumaker-Parry. have studied citrate and thiol co-adsorbed layer and their chemistry for functional gold nanoparticles[65]. Burrows, Nathan D., et al. have highlighted the chemistry of nanoparticles anisotropy, regiospecfic functionalization and also discussed surface chemistry of gold nanorods with an outlook for surface chemistry toolbox development of the synthetic nanoparticles[66,67]. Rossi, Liane M., et al. have reviewed the role of surface functionalization/capping of metal nanoparticles in catalysis with the perspective of surface tuning for the activity and selectivity[68]. Some researchers have highlighted polydopamine surface chemistry and primary, secondary functionalization role in biomedical applications[69,70]. Gao, Chuanbo, Fenglei Lyu, and Yadong Yin have discussed the encapsulation of metal nanoparticles in nanopores or nanoshells and their subsequent stabilization. They have also discussed encapsulation in nanoshells role catalysis in context of inorganic oxides, porous materials, organic frameworks, dendrimers, and organic cages[71]. Soriano, Gustavo Bonomi, et al. have used block copolymer to stabilize silver nanoparticles and studied their interactions with cellular membrane model[72]. M. Sahooli and S. Sabbaghi, have reported the stability and dispersion of CuO Nanoparticles in ethylene glycol-water mixture using pH, temperature and weight percent effects as the factors. They have studied the optimum properties when the nanoparticles are more dispersed and stabilized[73]. Shadpour Mallakpour and Atefeh Jarahiyan have reported the dispersion of CuO nanoparticles surface functionalized with ascorbic acid and citric acid. They have studied improved thermal properties for poly (vinyl alcohol)/CuO nanocomposites with well dispersed nanoparticles[74]. C. Karuppiah et al. have reported ZnO nanorods CuO nanospheres (ZnO-CuO) heterostructures. They have confirmed their formation through SEM, energy dispersive X-ray spectroscopy, X-ray diffraction and cyclic voltammetry[75]. Some researchers have stabilized and functionalized TiO2, ZnO, CeO2 Nanoparticles for transparent catalytic, UV-protecting coatings, sunscreens, thermomechanical properties improvement etc. with different additives[76-78]. Zhou, Li, et al. have reported the synthesis of organic functional group decorated molybdenum disulphide (MoS2 ) nanosheets by exploiting chemical conjugations of thiol ligands with MoS2 bulk crystals[79].
2.2 Silsesquioxane hybrids: role of silica core and organic periphery
Based on the siloxane core both silsesquioxane and silica have some similar physical properties, but the peripheral functional groups in silsesquioxane and one-half difference of oxygen in silsesquioxane structure allow for its different charging behaivour, ease of functionalization, colloidal stability, pH and temperature response. These fascinating properties of silsesquioxanes helpful in surface modification are reviewed here. Wang, Dan, et al. have chemically linked carbon dot carboxylic acid groups with the amine functional POSS to achieve composite filler with enhanced photoluminescence and thermal stability for sensing applications[80]. Dong, Fuping, Liangyu Lu, and Chang-Sik Ha, have highlighted the developments in POSS containing hybrid materials including their structures and chemical reactions in context of catalysis, polymer composites, adsorption, sensing and biomedical applications[81]. Sun, Di, Wei Wang, and Dan Yu. have reported alkyl functionalized POSS achieved via click-chemistry (photochemical thiol reaction), employed for cotton fabric super-hydrophobic modifications, fluorine free, durable and most effective with four dodecyl and four triethoxysilyl groups[82]. Przybylak, Marcin, Hieronim Maciejewski, and Agnieszka Dutkiewicz. have reported bifunctional fluorinated POSS prepared via sol-gel and dip coating methods, applied for cotton fabric hydrophobic modifications, and durability[83]. Wang, Wen-Jing, et al. have reported octa-aminopropyl POSS (OA-POSS) functionalized carbon dots for cell imaging. The amino groups on OA-POSS form amide bond with carboxylic groups on carbon dots to afford water soluble inorganic-organic hybrid carbon dots[16]. Ge, Qian, and Hongzhi Liu have reported amine-functionalized POSS as adsorbent for heavy metals removal via nucleophilic substitution of octa(3-chloropropyl) silsesquioxane with triaminoethylamine silsesquioxane. They have studied improved thermal stability and hydrophilicity for the as synthesized silsesquioxanes with an ease of regeneration and potential for water purification[84]. Zhang, R.L., et al. have reported the preparation of hierarchical carbon fiber achieved by the co-grafting of POSS and CNTs, and have investigated increased interfacial adhesion, improved mechanical performance for the as prepared co-grafted fiber-resin composite[85]. Yang, Xiaoru, and Hongzhi Liu have reported porous ferrocene functionalized POSS and porous pyrene-functionalized POSS via Friedel-Crafts reaction of octavinyl silsesquioxane with ferrocene and pyrene respectively. They have investigated regeneration and water purification performances of the as prepared functionalized POSS[86,87]. Chuo, Tsai-Wei, and Ying-Ling Liu. have utilized methacrylated-POSS and furfurylamine to achieve methacrylated furan Diels-Alder adducts in the composite structure exhibiting thermally stable self-healing ability and thermal reversibility[88]. Akbari, A., Naderahmadian, A., and Eftekhari-Sis, B. have reported preparation of catalysts using OA-POSS and ionic liquid followed by copper and silver nanoparticles immobilization for click reaction in aqueous medium and nitro-phenol reduction with easy recovery and recyclability[89]. Song, Shasha, et al. have investigated dispersion state, thermal conductivity and thermal stability of PVDF composite membranes incorporated with POSS functionalized CNTs[90]. Shi, Mengni, et al. employed gamma-radiation induce grafting under mild conditions to achieve functionalization of graphene oxide with octavinyl POSS. They have reported improved thermal stability and hydrophilicity with green chemistry approach[91]. Selvaraj and Alagar have stabilized and functionalized silver nanoparticles by amino-functionalized POSS, with diverse shaped nanoparticles for glucose oxidation by the chemical reduction of AgNO3 using glycerol as reducing agent[8]. Dell’Erba et al. have reported silver nanoparticles coated with OH-functionalized organic groups as nanofiller for different applications[1]. J. Zheng et al. have reported Ag Nanoparticles (Ag NPs) through common chemical reduction by interface regulation between AgNO3 and graphene oxide (GO) using hydrophobic POS[29]. Zhang, Jingyan, et al. have reported unique chain architecture of star shape polymers with outer cross-linking using octa(3-azidopropyl) POSS by click reaction[92].
3. Effect of Fillers Dispersion: Functional Polymer Composites
Homogeneous dispersion of nanoparticles (3D fillers, nanotubes and nanofibers, plate like fillers, silsesquioxanes, and metal/metal oxides) is the key step in nanotechnology industrialization and functional materials development. Different strategies adopted for homogeneous dispersion of fillers have been discussed and the schematic is shown in Figure 1.
Ag and Au nanoparticles can be dispersed by the reduction of organometallic compounds in polymer matrix for optical property modification[8,51,93-95]. They have reported the absorption spectra of Ag and Au film at different treatment duration[27]. Klaus Mullen have studied different dispersion dependent properties of the ZnO/PMMA transparent UV-protective nanocomposites. They have reported dispersion and re-dispersion in the toluene hydrophobic medium[96]. Zong, Peisong, et al. have achieved homogeneous dispersion and interfacial interactions by preparing aminopropylisobutyl POSS (Ap-POSS) covalently grafted with graphene. They have investigated that the as prepared graphene-Ap-POSS nonhybrids have interfacial interactions with epoxy polymer matrix through non-covalent and covalent bonds that promote dispersibility, compatibility in the composites as demonstrated by the improved thermal conductivity and decreased thermal interfacial resistance[97]. Liu, Konghua, et al. have reported homogeneously dispersed silver nanoparticles decorated graphene by utilizing poly(amidoamine) as stabilizing and reducing agent for the preparation of conducting polymer composites[98]. Ben-Sasson, Moshe, et al. have reported surface functionalization of polyimide reverse osmosis (RO) membrane with copper nanoparticles (Cu NPs) by employing the electrostatic interactions between polyethyleneimine capped positively charged Cu NPs and negatively charged polyimide RO membrane[99]. Kanahara, M., Shimomura, M., and Yabu, H. have fabricated gold nanoparticles (Au NPs) polymer composite particles by employing the electrostatic interactions between citrate stabilized Au NPs and amino-terminated polymer particles[100]. They have investigated the adsorption and diffusion of the Au NPs on the polymer particles based on glass transition temperature and molecular weights of the polymer particles. Huang, Qiang, et al. have reported the synthesis of molybdenum disulphide (MoS2 ) polymer composite by the self-polymerization of levodopa in weak alkaline medium on the surface of MoS2 for adsorption of methylene blue from aqueous solution[101].
The dispersion of fillers in functional polymer composites is a crucial factor that affects the mechanical, thermal, and electrical properties of the composite material. The homogeneous dispersion of nanoparticles such as 3D fillers, nanotubes, and nanofibers, platelike fillers, silsesquioxanes, and metal/metal oxides is essential to achieve the desired properties. Several studies have been conducted to explore practical examples demonstrating the homogeneous dispersion of these fillers. For instance, a study by Wang et al. demonstrated the homogeneous dispersion of carbon nanotubes in a polymer matrix using scanning electron microscopy (SEM) and transmission electron microscopy (TEM)[102]. Another study by Liu et al. investigated the dispersion of graphene oxide in a polymer matrix using SEM and TEM[103]. These studies provide valuable insights into the methods employed to achieve homogeneous filler dispersion in polymer composites.
The future is now open to the synergistic integration of nanocomposites with 3D printing, which is soon projected to eclipse its competition with conventional production methods. Preparing a nanocomposite resin for polymerization-based 3D printing, which involves the agglomeration of nanoparticles, has always been a challenge. The nanoparticles cluster in the composite matrix as a result of the high surface-area-to-volume ratio, which influences the final characteristics. In an effort to examine the mechanical and thermal properties of 3D-printed nanocomposites, Mussadiq et. al. dispersed graphene oxide (GO) in it. For this purpose, high and poor GO dispersions were analyzed along with their influence on various attributes using a well-dispersed sonication dispersion technique[104]. Microscopy was used to examine the fracture surfaces of the control and poorly and highly scattered printed composite tensile specimens. A typical microstructure of brittle materials, as reported in the literature[105,106], is shown in Figure 2
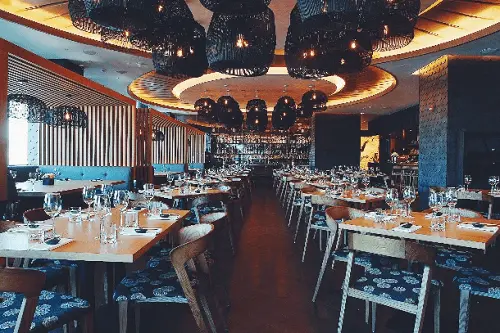
Figure 2. The fracture surface of (a, b) control specimens and (c, d) poorly dispersed and (e, f) highly dispersed nanocomposites[104].
Furthermore, gaps between the GO and the polymer matrix are also visible on the fracture surface, suggesting that the nanofiller–matrix interface is weak, which causes debonding and a multitude of river-like formations. The fractures occur after a failure that resembles brittleness but leans toward ductility[109]. There are additional gaps or black surfaces, which are also caused by the nanofiller aggregating inside the polymer matrix. This leads to inadequate mechanical characteristics and insufficient polymerization. The increase in GO loading further impacted the brittleness of printed nanocomposites in tensile testing. Consequently, the voids were the primary cause of the brittle behavior.
Consequently, the voids were the primary cause of the brittle behavior. As the tensile strength increases, these gaps allow abrupt fracture, leading to brittle fractured surfaces, as shown by[109]. When the stress surpasses the composite interface strength, the weak nanofiller–polymer matrix interface causes the GO to be pulled as the micro-cracks spread. The rough fracture surface is proof that the exfoliation or pullout of GO encourages local plastic deformation of the polymer matrix to release the fracture energy. Comparably, as seen in Figure 2
4. Conclusions and Future Outlook
This perspective discusses the synthesis and role of functional fillers for polymer composites, with a focus on their dispersion, stability, compatibility, non-covalent, and covalent interactions. The performance of the functional polymer composites is mainly influenced by the polymer matrix's porosity, general thermomechanical stability, and nanoparticle dispersion within the nanocomposites. The stable dispersion of the nanoparticles with intrinsic characteristics intact in the functional polymer composites will be necessary for these materials to be commercialized in the future. Functional metal/metal oxide nanofillers or POSS nanocages, which have favorable biocompatibility, non-toxicity, and cyto-compatibility, could be used as desired nanocarrier candidates to encapsulate hydrophobic drugs into self-assembled cavities, resulting in improved aqueous solubility and a controlled drug release profile. Similarly, POSS-based complex systems for gene therapy have been developed by numerous research groups with the goal of increasing transfection efficiency. The successful application of POSS-based hybrid materials in biomedical imaging plays a crucial role in the diagnosis/detection of diseases and the assessment of therapeutic effect due to the superior physicochemical properties (such as excellent biocompatibility and simple design for surface multifunctionalities). Due to their strong biocompatibility, osteo-conductivity, and osteoinductivity, silica-based nanoparticles have shown promise in clinical use and for bone tissue restoration. As compared to ordinary nanomaterials, POSS-incorporated hydrogels displayed a homogeneous system without a phase boundary, which resulted in increased structural stability when exposed to external stress. They may also serve as an inorganic cross-linker in an otherwise organic hydrogel network due to their versatility, which helped to firmly anchor them in the network and improve its properties through molecularly controlled mechanisms. Numerous investigations have demonstrated that POSS-incorporated hybrid hydrogels were cytocompatible and facilitated chondrocyte adhesion, dissemination, and proliferation.
Declarations
Author contributions
Muhammad S: Writing the original draft and edited.
Batool F: Writing the original draft and edited.
Irfan M: Formal analysis.
Ahmad M:Formal analysis.
Conflicts of interest
The authors declare no conflict of interest.
Ethical approval
Not applicable.
Consent to participate
Not applicable
Consent for publication
Not applicable.
Availability of data and materials
Not applicable.
Funding information
None.
Copyright
© The Author(s) 2023.
References
-
1. Erba IE, Hoppe CE, Williams RJ. Synthesis of silver nanoparticles coated with OH-functionalized organic groups: dispersion and covalent bonding in epoxy networks. Langmuir. 2010;26(3):2042-2049.
[DOI] -
2. Zhou J, Ralston J, Sedev R, Beattie DA. Functionalized gold nanoparticles: synthesis, structure and colloid stability. J Colloid Interface Sci. 2009;331(2):251-62.
[PubMed] -
3. Carroll JB, Frankamp BL, Rotello VM. Self-assembly of gold nanoparticles through tandem hydrogen bonding and polyoligosilsequioxane (POSS)–POSS recognition processes. Chem Commun. 2002(17): 1892-1893;
[DOI] -
4. Wang ZL. Zinc oxide nanostructures: growth, properties and applications. J Phys: Condens Matter. 2004;16(25):R829-R858.
[DOI] -
5. Laurent S, Forge D, Port M, Roch A, Robic C, Vander Elst, et al. Magnetic iron oxide nanoparticles: synthesis, stabilization, vectorization, physicochemical characterizations, and biological applications. Chem Rev. 2008;108(6):2064-110. doi: 10.1021/cr068445e. Erratum in: Chem Rev. 2010 Apr 14;110(4):2574-2574.
[PubMed] -
6. Cheetham AK, Rao C, Feller RK. Structural diversity and chemical trends in hybrid inorganic–organic framework materials. Chem Commun. 2006(46):4780-4795;
[DOI] -
7. Schubert U, Huesing N, Lorenz A. Hybrid inorganic-organic materials by sol-gel processing of organofunctional metal alkoxides. Chem of Mater. 1995;7(11):2010-2027.
[DOI] -
8. Selvaraj V, Alagar M. Synthesis of Novel Ag/POSS Nanocomposite Powder for Glucose Oxidation and Antimicrobial Applications. J Bionanosci. 2008;2(1):54-61.
[DOI] -
9. Cai J, Lv C, Watanabe A. Facile Preparation of Hierarchical Structures Using Crystallization-Kinetics Driven Self-Assembly. ACS Appl Mater Interfaces. 2015;7(33):18697-18706.
[DOI] -
10. Hwang SJ, Jeng SC, Yang CY, Kuo CW, Liao CC. Characteristics of nanoparticle-doped homeotropic liquid crystal devices. J Phys D Appl Phys. 2009;42:025102-025102.
[DOI] -
11. Ullah A, Ullah S, Khan GS, Shah SM, Hussain Z, Muhammad S, et al. Water soluble polyhedral oligomeric silsesquioxane based amphiphilic hybrid polymers: Synthesis, self-assembly, and applications. Eur Polym J. 2016;75:67-92.
[DOI] -
12. Feng X, Zhu S, Yue K, Su H, Guo K, Wesdemiotis C, et al. T10 Polyhedral Oligomeric Silsesquioxane-Based Shape Amphiphiles with Diverse Head Functionalities via "Click" Chemistry. ACS Macro Lett. 2014;3(9):900-905.
[DOI] -
13. Kannan RY, Salacinski HJ, De Groot, Clatworthy I, Bozec L, Horton M, et al. The antithrombogenic potential of a polyhedral oligomeric silsesquioxane (POSS) nanocomposite. Biomacromolecules. 2006;7(1):215-223.
[DOI] -
14. Engstrand J, López A, Engqvist H, Persson C. Polyhedral oligomeric silsesquioxane (POSS)-poly(ethylene glycol) (PEG) hybrids as injectable biomaterials. Biomed Mater. 2012;7(3):035013-035013.
[DOI] -
15. Ayandele E, Sarkar B, Alexandridis P. Polyhedral Oligomeric Silsesquioxane (POSS)-Containing Polymer Nanocomposites. Nanomaterials (Basel). 2012;2(4):445-475.
[DOI] -
16. Wang WJ, Hai X, Mao QX, Chen ML, Wang JH. Polyhedral Oligomeric Silsesquioxane Functionalized Carbon Dots for Cell Imaging. ACS Appl Mater Interfaces. 2015;7(30):16609-16616.
[DOI] -
17. Wang D, Liu JY, Chen J-F, Dai LM. Surface Functionalization of Carbon Dots with Polyhedral Oligomeric Silsesquioxane (POSS) for Multifunctional Applications. Advanced Materials Interfaces. 2016;3(1):1500439-1500439.
[DOI] -
18. Rahman MM, Filiz V, Khan MM, Gacal BN, Abetz V. Functionalization of poss nanoparticles and fabrication of block copolymer nanocomposite membranes for CO2 separation. React Funct Polym. 2015;86:125-133.
[DOI] -
19. Ghanbari H, Cousins BG, Seifalian AM. A nanocage for nanomedicine: polyhedral oligomeric silsesquioxane (POSS). Macromol Rapid Commun. 2011;32(14):1032-46.
[DOI] -
20. Tishchenko G, Bleha M. Diffusion permeability of hybrid chitosan/polyhedral oligomeric silsesquioxanes (POSSTM) membranes to amino acids. J Membr Sci. 2005;248(1-2):45-51.
[DOI] -
21. Wu J, Mather PT. POSS Polymers: physical properties and biomaterials applications. Polym Rev. 2009;49(1):25-63.
[DOI] -
22. Sanchez C, Belleville P, Popall M, Nicole L. Applications of advanced hybrid organic–inorganic nanomaterials: from laboratory to market. Chem Soc Rev. 2011;40(2):696-753.
[DOI] -
23. Sanchez C, Shea KJ, Kitagawa S. Recent progress in hybrid materials science. Chem Soc Rev. 2011;40(2):471-472.
[DOI] -
24. Mbhele ZH, Salemane MG, van Sittert, Nedeljković JM, Djoković V, Luyt AS. Fabrication and characterization of silver - polyvinyl alcohol nanocomposites. Chem Mater. 2003;15(26):5019-5024.
[DOI] -
25. Blanco I, Abate L, Bottino FA. Variously substituted phenyl hepta cyclopentyl-polyhedral oligomeric silsesquioxane (ph,hcp-POSS)/polystyrene (PS) nanocomposites. J Therm Anal Calorim. 2013;112:421-428.
[DOI] -
26. Mu J, Zheng S. Poly(N-isopropylacrylamide) nanocrosslinked by polyhedral oligomeric silsesquioxane: temperature-responsive behavior of hydrogels. J Colloid Interface Sci. 2007;307(2):377-85.
[DOI] -
27. M , Martynková GS, Barabaszová K. Effect of nanofillers dispersion in polymer matrices: a review. Sci Adv Mater. 2011;3(1):1-25.
[DOI] -
28. Selvaraj V, Grace AN, Jothibasu S, Nagendiran S, Alagar M. Synthesis and characterization of Au/POSS composite powder for bio-fuel cells and antibiotic applications. J Nanosci Nanotechnol. 2009;9(10):5997-6002.
[DOI] -
29. Bai WS, Sheng QL, Ma XY, Zheng JB. Synthesis of silver nanoparticles based on hydrophobic interface regulation and its application of electrochemical catalysis. ACS Sustainable Chem Eng. 2015;3(7):1600-1609.
[DOI] -
30. Létant SE, Herberg J, Dinh LN, Maxwell RS, Simpson RL, Saab AP. Structure and catalytic activity of POSS-stabilized Pd nanoparticles. Catal Commun. 2007;8(12):2137-2142.
[DOI] -
31. Aflori M, Simionescu B, Bordianu IE, Sacarescu L, Varganici CD, Doroftei F, et al. Silsesquioxane-based hybrid nanocomposites with methacrylate units containing titania and/or silver nanoparticles as antibacterial/antifungal coatings for monumental stones. Mater Sci Eng: B. 2013;178(19):1339-1346.
[DOI] -
32. Lu C-H, Chang F-C. Polyhedral oligomeric silsesquioxane-encapsulating amorphous palladium nanoclusters as catalysts for Heck reactions. ACS Catal. 2011;1(5):481-488.
[DOI] -
33. Liu YZ, Sun Y, Zeng FL, Liu JC, Ge JR. Effect of POSS nanofiller on structure, thermal and mechanical properties of PVDF matrix. J Nanopart Res. 2013;15:2116-2116.
[DOI] -
34. Fina A, Abbenhuis HCL, Tabuani D, Frache A, Camino G. Polypropylene metal functionalised POSS nanocomposites: a study by thermogravimetric analysis. Polym Degrad and Stabil. 2006;91(5):1064-1070.
[DOI] -
35. Fu H-K, Kuo S-W, Yeh D-R, Chang F-C. Properties enhancement of PS nanocomposites through the POSS surfactants. J Nanomater. 2008;2008:739613-739613.
[DOI] -
36. Qian Y, Wei P, Zhao XM, Jiang PK, Yu HZ. Flame retardancy and thermal stability of polyhedral oligomeric silsesquioxane nanocomposites. Fire Mater. 2013;37(1):1-16.
[DOI] -
37. Matai I, Sachdev A, Dubey P, Kumar SU, Bhushan B, Gopinath P. Antibacterial activity and mechanism of Ag-ZnO nanocomposite on S. aureus and GFP-expressing antibiotic resistant E. coli. Colloids Surf B Biointerfaces. 2014;115:359-67.
[DOI] -
38. Sanchez C, Lebeau B, Chaput F, Boilot J-P. Optical properties of functional hybrid organic-inorganic nanocomposites. Adv Mater. 2003;15(23):1969-1994.
[DOI] -
39. Zhang ZP, Gu AJ, Liang GZ, Ren PG, Xie JQ, Wang XL. Thermo-oxygen degradation mechanisms of POSS/epoxy nanocomposites. Polym Degrad Stabil. 2007;92(11):1986-1993.
[DOI] -
40. Kuo S-W, Chang F-C. POSS related polymer nanocomposites. Prog Polym Sci. 2011;36(12):1649-1696.
[DOI] -
41. Fina A, Bocchini S, Camino G. Catalytic fire retardant nanocomposites. Polym Degrad Stabil. 2008;93(9):1647-1655.
[DOI] -
42. Folarin OM, Sadiku ER. Maity A. Polymer-noble metal nanocomposites: review. Int J Phys Sci. 2011;6(21):4869-4882. Available from: https://citeseerx.ist.psu.edu/document?repid=rep1&type=pdf&doi=a507d587a4d4f5b556947d8270f43903c324fb72
-
43. Faure B, Salazar-Alvarez G, Ahniyaz A, Villaluenga I, Berriozabal G, De Miguel, et al. Dispersion and surface functionalization of oxide nanoparticles for transparent photocatalytic and UV-protecting coatings and sunscreens. Sci Technol Adv Mater. 2013;14(2):023001-023001.
[DOI] -
44. Chen Y, Xianyu Y, Jiang X. Surface Modification of Gold Nanoparticles with Small Molecules for Biochemical Analysis. Acc Chem Res. 2017;50(2):310-319.
[DOI] -
45. Kango S, Kalia S, Celli A, Njuguna J, Habibi Y, Kumar R. Surface modification of inorganic nanoparticles for development of organic–inorganic nanocomposites - a review. Prog Polym Sci. 2013;38(8):1232-1261.
[DOI] -
46. Muhammad S, Siddiq M, Niazi JH, Qureshi A. Role of quaternary ammonium compound immobilized metallic graphene oxide in PMMA/PEG membrane for antibacterial, antifouling and selective gas permeability properties. Polym Bull. 2018;75:5695-5712.
[DOI] -
47. Muhammad S, Niazi JH, Qureshi A. Silver nanoparticles decorated polyhedral oligomeric silsesquioxane nanocages as an effective nanoadditive for improved structural and biological properties of poly (vinylidene fluoride-co-hexafluoropropylene) nanofiltration membrane. Mater Today Chem. 2022;23:100643-100643.
[DOI] -
48. Lei C, Zhou Z, Chen W, Xie J, Huang B. Polypyrrole supported Pd/Fe bimetallic nanoparticles with enhanced catalytic activity for simultaneous removal of 4-chlorophenol and Cr(VI). Sci Total Environ. 2022;831:154754-154754.
[DOI] -
49. Shrestha S, Wang B, Dutta P. Nanoparticle processing: Understanding and controlling aggregation. Adv Colloid Interface Sci. 2020;279:102162-102162.
[DOI] -
50. Gupta AK, Gupta M. Synthesis and surface engineering of iron oxide nanoparticles for biomedical applications. Biomaterials. 2005;26(18):3995-4021.
[DOI] -
51. Castelvetro V, De Vita. Nanostructured hybrid materials from aqueous polymer dispersions. Adv Colloid Interface Sci. 2004;108-109:167-185.
[DOI] -
52. Griffete N, Clift MJD, Lamouri A, Digigow RG, Mihut AM, Fink A, et al. Amino covalent binding approach on iron oxide nanoparticle surface: toward biological applications. Colloid Surface A. 2012;415:98-104.
[DOI] -
53. Maity D, Agrawal DC. Synthesis of iron oxide nanoparticles under oxidizing environment and their stabilization in aqueous and non-aqueous media. J Magn Magn Mater. 2007;308(1):46-55.
[DOI] -
54. Qiao R, Esser L, Fu C, Zhang C, Hu J, Ramírez-Arcía P, et al. Bioconjugation and Fluorescence Labeling of Iron Oxide Nanoparticles Grafted with Bromomaleimide-Terminal Polymers. Biomacromolecules. 2018;19(11):4423-429.
[DOI] -
55. Hola K, Markova Z, Zoppellaro G, Tucek J, Zboril R. Tailored functionalization of iron oxide nanoparticles for MRI, drug delivery, magnetic separation and immobilization of biosubstances. Biotechnol Adv. 2015;33(6):1162-1176.
[DOI] -
56. Lee SH, Nishi H, Tatsuma T. Tunable plasmon resonance of molybdenum oxide nanoparticles synthesized in non-aqueous media. Chem Commun. 2017;53(94):12680-12683.
[DOI] -
57. Patil RM, Shete PB, Thorat ND, Otari SV, Barick KC, Prasad A, et al. Non-aqueous to aqueous phase transfer of oleic acid coated iron oxide nanoparticles for hyperthermia application. RSC Adv. 2014;4(9):4515-4522.
[DOI] -
58. Alliraja C, Rao JR, Thanikaivelan P. Magnetic collagen fibers stabilized using functional iron oxide nanoparticles in non-aqueous medium. RSC Adv. 2015;5(27):20939-20944.
[DOI] -
59. Kharisov BI, Dias HVR, Kharissova OV, Vázquez A, Peña Y, Gómez I. Solubilization, dispersion and stabilization of magnetic nanoparticles in water and non-aqueous solvents: recent trends. RSC Adv. 2014;4(85):45354-45381.
[DOI] -
60. Shankar R, Groven L, Amert A, Whites KW, Kellar JJ. Non-aqueous synthesis of silver nanoparticles using tin acetate as a reducing agent for the conductive ink formulation in printed electronics. J Mater Chem. 2011;21(29):10871-10877.
[DOI] -
61. Peiris TAN, Weerasinghe HC, Sharma M, Kim J-E, Michalska M, Chandrasekaran N, et al. Non-aqueous one-pot SnO2 nanoparticle inks and their use in printable perovskite solar cells. Chem Mater. 2022;34(12):5535-5545.
[DOI] -
62. Kharissova, O.V., B.I. Kharisov, and E.G. de Casas Ortiz. Dispersion of carbon nanotubes in water and non-aqueous solvents. RSC Adv. 2013;3(47):24812-24852..Available from: https://www.researchgate.net/publication/260204417_Dispersion_of_carbon_nanotubes_in_water_and_non-aqueous_solvents
-
63. Kaushik M, Moores A. Review: nanocelluloses as versatile supports for metal nanoparticles and their applications in catalysis. Green Chem. 2016;18(3):622-637.
[DOI] -
64. Wang S, McGuirk CM, d'Aquino A, Mason JA, Mirkin CA. Metal-Organic Framework Nanoparticles. Adv Mater. 2018;30(37):e1800202-e1800202.
[DOI] -
65. Park JW, Shumaker-Parry JS. Strong resistance of citrate anions on metal nanoparticles to desorption under thiol functionalization. ACS Nano. 2015;9(2):1665-1682.
[DOI] -
66. Burrows ND, Vartanian AM, Abadeer NS, Grzincic EM, Jacob LM, Lin W, et al. Anisotropic Nanoparticles and Anisotropic Surface Chemistry. J Phys Chem Lett. 2016;7(4):632-641.
[DOI] -
67. Burrows ND, Lin W, Hinman JG, Dennison JM, Vartanian AM, Abadeer NS, et al. Surface Chemistry of Gold Nanorods. Langmuir. 2016;32(39):9905-9921.
[DOI] -
68. Rossi LM, Fiorio JL, Garcia MAS, Ferraz CP. The role and fate of capping ligands in colloidally prepared metal nanoparticle catalysts. Dalton Trans. 2018;47(17):5889-5915.
[DOI] -
69. Ding YH, Floren M, Tan W. Mussel-inspired polydopamine for bio-surface functionalization. Biosurf Biotribol. 2016;2(4):121-136.
[DOI] -
70. Ryu JH, Messersmith PB, Lee H. Polydopamine Surface Chemistry: A Decade of Discovery. ACS Appl Mater Interfaces. 2018;10(9):7523-7540.
[DOI] -
71. Gao C, Lyu F, Yin Y. Encapsulated Metal Nanoparticles for Catalysis. Chem Rev. 2021;121(2):834-881.
[DOI] -
72. Soriano GB, da Silva, Camilo FF, Caseli L. Interaction of non-aqueous dispersions of silver nanoparticles with cellular membrane models. J Colloid Interface Sci. 2017;496:111-117.
[DOI] -
73. Sahooli M, Sabbaghi S. Investigation of thermal properties of CuO nanoparticles on the ethylene glycol - water mixture. Mater Lett. 2013;93:254-257.
[DOI] -
74. Mallakpour S, Jarahiyan A. An eco-friendly approach for the synthesis of biocompatible poly(vinyl alcohol) nanocomposite with aid of modified CuO nanoparticles with citric acid and vitamin C: mechanical, thermal and optical properties. J Iran Chem Soc. 2016;13:509-518.
[DOI] -
75. Karuppiah, C., Velmurugan, M., Chen, S.M., et al. A simple hydrothermal synthesis and fabrication of zinc oxide - copper oxide heterostructure for the sensitive determination of nonenzymatic glucose biosensor. Sens Actuators B. 2015;221:1299-1306.
[DOI] -
76. Faure B, Salazar-Alvarez G, Ahniyaz A, Villaluenga I, Berriozabal G, De Miguel, et al. Dispersion and surface functionalization of oxide nanoparticles for transparent photocatalytic and UV-protecting coatings and sunscreens. Sci Technol Adv Mater. 2013;14(2):023001-023001.
[DOI] -
77. Nguyen VS, Rouxel D, Vincent B, Badie L, Dos Santos, Lamouroux E, et al. Influence of cluster size and surface functionalization of ZnO nanoparticles on the morphology, thermomechanical and piezoelectric properties of P (VDF-TrFE) nanocomposite films. Appl Surf Sci. 2013;279:204-211.
[DOI] -
78. Mallakpour S, Madani M. Use of silane coupling agent for surface modification of zinc oxide as inorganic filler and preparation of poly(amide-imide)/zinc oxide nanocomposite containing phenylalanine moieties. Bull Mater Sci. 2012;35:333-339.
[DOI] -
79. Zhou L, He B, Yang Y, He Y. Facile approach to surface functionalized MoS2 nanosheets. RSC Adv. 2014;4(61):32570-32578.
[DOI] -
80. Wang D, Liu J, Chen J-F, Dai L. Surface functionalization of carbon dots with polyhedral oligomeric silsesquioxane (POSS) for multifunctional applications. Adv Mater Interf. 2016;3(1):1500439-1500439.
[DOI] -
81. Dong F, Lu L, Ha C-S. Silsesquioxane‐containing hybrid nanomaterials: fascinating platforms for advanced applications. Macromol Chem Phys. 2019;220(3):1800324-1800324.
[DOI] -
82. Sun D, Wang W, Yu D. Highly hydrophobic cotton fabrics prepared with fluorine-free functionalized silsesquioxanes. Cellulose. 2017;24:4519-4531.
[DOI] -
83. Przybylak M, Maciejewski H, Dutkiewicz A. Preparation of highly hydrophobic cotton fabrics by modification with bifunctional silsesquioxanes in the sol-gel process. Appl Surf Sci. 2016;387:163-174.
[DOI] -
84. Ge Q, Liu H. Tunable amine-functionalized silsesquioxane-based hybrid networks for efficient removal of heavy metal ions and selective adsorption of anionic dyes. Chem Eng J. 2022;428:131370-131370.
[DOI] -
85. Zhang RL, Wang CG, Liu L, Cui HZ, Gao B. Polyhedral oligomeric silsesquioxanes/carbon nanotube/carbon fiber multiscale composite: Influence of a novel hierarchical reinforcement on the interfacial properties. Appl Surf Sci. 2015;353:224-231.
[DOI] -
86. Yang X, Liu H. Ferrocene-functionalized silsesquioxane-based porous polymer for efficient removal of dyes and heavy metal ions. Chemistry. 2018;24(51):13504-13511.
[DOI] -
87. Yang H, Liu H. Pyrene-functionalized silsesquioxane as fluorescent nanoporous material for antibiotics detection and removal. Microporous Mesoporous Mater. 2020;300:110135-110135.
[DOI] -
88. Chuo T-W, Liu Y-L. Preparation of self-healing organic–inorganic nanocomposites with the reactions between methacrylated polyhedral oligomeric silsesquioxanes and furfurylamine. Compos Sci Technol. 2015;118:236-243.
[DOI] -
89. Akbari A, Naderahmadian A, Eftekhari-Sis B. Silver and copper nanoparticles stabilized on ionic liquids-functionalized polyhedral oligomeric silsesquioxane (POSS): highly active and recyclable hybrid catalysts. Polyhedron. 2019;171:228-236.
[DOI] -
90. Song S, Cao M, Shan H, Du C, Li B. Polyhedral oligomeric silsesquioxane functionalized carbon nanotubes for high thermal conductive poly (vinylidene fluoride) composite membrane. Mater Des. 2018;156:242-251.
[DOI] -
91. Shi M, Lin T, Hu Y, Peng J, Li J, Zhai M. Functionalization of graphene oxide by radiation grafting polyhedral oligomeric silsesquioxane with improved thermal stability and hydrophilicity. J Mater Sci. 2020;55:1489-1498.
[DOI] -
92. Zhang J, Si D, Wang S, Liu H, Chen X, Zhou H, et al. Novel organic/inorganic hybrid star polymer surface-crosslinked with polyhedral oligomeric silsesquioxane. Macromol Res. 2020;28:152-158.
[DOI] -
93. Ogawa S, Hayashi Y, Kobayashi N, Tokizaki T, Nakamura A. Novel preparation method of metal particles dispersed in polymer films and their third-order optical nonlinearities. Jpn J Appl Phys. 1994;33(3A):L331-L331.
[DOI] -
94. Pomogailo AD, Dzhardimalieva GI. Reduction of metal ions in polymer matrices as a condensation method of nanocomposite synthesis. In: Nanostructured Materials Preparation via Condensation Ways. Springer. Dordrecht;
[DOI] -
95. Hakemi HA, inventor; Sniaricerche SCpA, assignee. Metallo organo liquid crystals in a polymer matrix. Google Patents US5843333A. 1998.
-
96. Khrenov V, Klapper M, Koch M, Müllen K. Surface functionalized ZnO particles designed for the use in transparent nanocomposites. Macromol Chem Phys. 2005;
[DOI] -
97. Zong P, Fu J, Chen L, Yin J, Dong X, Yuan S, et al. Effect of aminopropylisobutyl polyhedral oligomeric silsesquioxane functionalized graphene on the thermal conductivity and electrical insulation properties of epoxy composites. RSC Adv. 2016;6(13):10498-10506.
[DOI] -
98. Liu K, Chen S, Luo Y, Jia D, Gao H, Hu G, et al. Noncovalently functionalized pristine graphene/metal nanoparticle hybrid for conductive composites. Compos Sci Technol. 2014;94:1-7.
[DOI] -
99. Ben-Sasson M, Zodrow KR, Genggeng Q, Kang Y, Giannelis EP, Elimelech M. Surface functionalization of thin-film composite membranes with copper nanoparticles for antimicrobial surface properties. Environ Sci Technol. 2014;48(1):384-393.
[DOI] -
100. Kanahara M, Shimomura M, Yabu H. Fabrication of gold nanoparticle-polymer composite particles with raspberry, core-shell and amorphous morphologies at room temperature via electrostatic interactions and diffusion. Soft Matter. 2014;10(2):275-280.
[DOI] -
101. Huang Q, Liu M, Chen J, Wan Q, Tian J, Huang L, et al. Facile preparation of MoS2 based polymer composites via mussel inspired chemistry and their high efficiency for removal of organic dyes. Appl Surf Sci. 2017;419:35-44.
[DOI] -
102. Xie X-L, Mai Y-W, Zhou X-P. Dispersion and alignment of carbon nanotubes in polymer matrix: a review. Mat Sci Eng R. 2005;49(4):89-112.
[DOI] -
103. Liu Q, Zhao Y, Gao S, Yang X, Fan R, Zhi M, et al. Recent advances in the flame retardancy role of graphene and its derivatives in epoxy resin materials. Composites. Part A;
[DOI] -
104. Shah M, Ullah A, Azher K, Ur Rehman, Akturk N, Juan W, et al. The influence of nanoparticle dispersions on mechanical and thermal properties of polymer nanocomposites using SLA 3D printing. Crystals. 2023;13(2):285-285.
[DOI] -
105. Gao H, Qiang T. Fracture Surface Morphology and Impact Strength of Cellulose/PLA Composites. Materials (Basel). 2017;10(6):624-624.
[DOI] -
106. Zhang S, Li M, Hao N, Ragauskas AJ. Stereolithography 3D printing of lignin-reinforced composites with enhanced mechanical properties. ACS Omega. 2019;4(23):20197-20204.
[DOI] -
107. Vidakis N, Petousis M, Michailidis N, Kechagias JD, Mountakis N, Argyros A, et al. High-performance medical-grade resin radically reinforced with cellulose nanofibers for 3D printing. J Mech Behav Biomed Mater. 2022;134:105408-105408.
[DOI] -
108. Dong D, Su H, Li X, Fan G, Zhao D, Shen Z, et al. Microstructures and mechanical properties of biphasic calcium phosphate bioceramics fabricated by SLA 3D printing. J Manuf Process. 2022;81:433-443.
[DOI] -
109. Markandan K, Lai CQ. Enhanced mechanical properties of 3D printed graphene-polymer composite lattices at very low graphene concentrations. Composites. Part A;
[DOI]
Copyright
© The Author(s) 2023. This is an Open Access article licensed under a Creative Commons Attribution 4.0 International License ( https://creativecommons.org/licenses/by/4.0/ ), which permits unrestricted use, sharing, adaptation, distribution and reproduction in any medium or format, for any purpose, even commercially, as long as you give appropriate credit to the original author(s) and the source, provide a link to the Creative Commons license, and indicate if changes were made.
Share And Cite