Abstract
The success of dental operations with the use of implants mostly depends on the properties of the implant materials and their compatibility with the tissues of the human body. A number of physical compatibility criteria are known, which include biological stability, biocorrosion resistance, chemical stability, antimicrobial resistance, so an in-depth study of these criteria is relevant. Among the used implants, dielectric materials of natural and synthetic origin make up a large share. The peculiarity of such materials, in contrast to metal ones, is the ability to form, at a human body temperature of 310K, low-intensity electromagnetic radiation (EMR) of the microwave range, which interacts with the biotissue adjacent to the implant. Such EMR exerts an influence at the cellular level too. This effect of EMRin fact has not been investigated. The article discusses the physical basis of the formation of positive ("+") and negative ("-") microwave radiation flows that occur between the implant and the adjacent biotissue. By using a highly sensitive radiometric system, an experimental study of the radiation properties of the most used implant materials contacting with human body tissues was carried out. In the course of the research the levels of inherent radiation of a number of dental materials were determined, the calculation and evaluation of the coefficients of their radiation capacity was carried out. Comparing the radiation capacity of contacting dental materials with the level of radiation of the human body tissues made it possible to determine the level of their electromagnetic compatibility. Recommendations for the selection of the most used contacting dental materials based on the criterion of electromagnetic compatibility have been developed.
Keywords
1. Introduction
Electromagnetic radiation (EMR) in the millimeter wavelength range is a general property of heated dielectrics. Dielectric materials include bone tissue of the human body, liquid fractions of blood and plasma, orthopedic and dental implants, as well as combined implants with a metal base and a dielectric coating[1-3]. This coating increases the biocompatibility of implants while preserving their physical and mechanical properties. At the same time, new contacting materials for the needs of dentistry are being researched, for example, based on resins, new combinations of dielectric materials for dental applications are being created using nanomaterials and bioengineering technologies[4].
The EMF power of dielectric materials at the temperature of the human body is insignificant, but due to the difference in the radiation of two contacting objects (implant and biotissue), negative ("-") or positive ("+") microwave energy flows can occur between them. These flows can have different directions and affect human tissues adjacent to the implant in a certain way. The body's reaction to this level of low-intensity signals is confirmed by laboratory studies and the practical application of low-intensity microwave therapy[5].
Dental filling materials have certain features of contact with living tissues. They are in contact with the hard tissues of the tooth, and may also partially have contact with periodontal tissues and saliva. Accordingly, the effect of emerging EMF flows is more difficult to detect and investigate. More important from this point of view are the processes occurring during the interaction of implanted materials and surrounding tissues.
Facial bone defects caused by injuries, wounds, inflammatory processes, tumors, etc., require complex reconstructive and restorative operations with a long rehabilitation period. The use of implanted contacting materials compatible by many criteria, including physical compatibility, creates conditions for reducing postoperative complications, shortening rehabilitation time and improving the patient's life[6,7].
Based on modern advances in tissue bioengineering, existing surgical methods for directed restoration of bones and soft tissues widely use biomimetic framework systems, both of synthetic and natural origin or their combination. The internal basis of such systems is a metal, ceramic or polymer frame, and the external coating is a dielectric[6-10].
The use of implanted materials for the purpose of eliminating facial bone defects involves their long-term stay in the human body under the influence of cyclic biomechanical loads in contact with the bone and soft tissues, including the mucous membrane[6,10]. It is expected that the implant materials will promote healing and tissue regeneration processes that are inevitably associated with inflammatory reactions. But at the same time, other reactions may occur (resorption and desorption of bone (the substance is released from the surface or through it), blood coagulation, suppuration, fibrous encapsulation, etc.), depending on the state of the implant bed. chemical and physical properties of materials, their destruction and/or biodegradation.
Problems arise constantly as "normal biology" becomes "abnormal" in direct contact with foreign materials transplanted in vivo. The so-called "smart surface" of implants looks quite static compared to the dynamic biology of a living organism.
Among the requirements for installing an implant and monitoring its functioning, an important place is occupied by the biophysical properties of implant materials, namely: high radiopacity, low magnetic susceptibility, strength (similar to or greater than that of adjacent living tissue), plasticity, surface structure, close coefficients of electrical conductivity and thermal conductivity, close levels of electromagnetic activity: emission and absorption of electromagnetic waves of a certain range.
At the same time, the level of electromagnetic radiation, its impact on organs, tissues and cells in the area of their contact with implanted materials remains the least studied criterion of their biophysical compatibility. Therefore, the study of the interaction of low-intensity microwave radiation of implanted materials, including biomedical materials, with the tissues of the human body, is an urgent task for specialists in various fields - biologists, doctors, physicists, developers of radio technical devices and instrument engineering.
The aims of this research are: measurement of electromagnetic radiation of the most used contacting dental materials; determination of emissivity coefficients of contacting dental materials and their comparison with the emissivity of biotissues of the human body; consideration of the physical processes of the emergence and formation of microwave electromagnetic fields and radiation at the boundary of the distribution of implant - adjacent biotissue; substantiation of assessment of electromagnetic compatibility of contact implanted materials with tissues of the human body and further experimental studies
2. Classification Features of Biomaterials
The use of biomaterials is associated with long-term studies and checks for compatibility with human biotissues The main indicators that are paid attention to are biological resistance, resistance to biocorrosion, chemical stability, antimicrobial resistance.This fully applies to all types of implant materials. The authors[11] proposed to supplement the known classification of requirements for biomaterials with the parameter of electromagnetic compatibility, as shown in Figure 1.
The criterion of electromagnetic compatibility applies to dielectric materials of natural and synthetic origin, as well as metal structures with a dielectric coating. Determination of electromagnetic compatibility, along with other parameters and requirements for biomaterials, can be one of the important stages of selection of known materials for the manufacture of more compatible implants in clinical conditions. In addition, the criterion of electromagnetic compatibility can be taken into account at earlier stages in the processes of development, testing and use of new implant materials, including those made with the use of nanomaterials and bioengineering technologies.
3. Peculiarities of Electromagnetic Interaction of Implants with Biotissue (Theoretical Part)
Studies of regulatory and adaptive processes of biological systems show that an integral part of the functioning of the human body, as well as intercellular interaction, is the presence of biochemical reactions. In turn, such biochemical reactions are accompanied by electromagnetic radiation and the formation of electromagnetic fields (EMF). Endogenous constant and variable electric fields, magnetic fields created by the heart, brain, muscles, bone tissue and all living cells are determined.
Liquid crystalline collagen is considered an important element of intercellular interaction at the molecular level, which is able to perceive and absorb EMR. By forming cluster systems with water molecules, collagen gives the connective tissue liquid crystalline properties, which facilitates the passage of EMF energy and can act as a communication system.
Connective tissue, along with the nervous and immune systems, hormones and biologically active substances, provides integrative and regulatory functions of the body. Signaling in this tissue can influence (or be influenced by) physiological or pathological processes, altering the state of health and the course of disease. It mainly forms a unique system - the electromagnetic homeostasis of the human body[12,13].
Numerous theoretical and experimental studies have been conducted on the influence of weak electromagnetic fields and radiation on the surface layers of biotissue, but the criterion of electromagnetic compatibility of biomaterials for implantation has not yet been applied.
Dielectric and combined materials used as intratissue implants, at the temperature of the human body, can form and/or change their own EMFs, which interact with the EMFs of the human body in the area of their introduction.The specified energy processes that occur during the electromagnetic interaction of the human body with biomaterial can be manifested at the local or general level of the organism and reflect the presence and functioning of the electromagnetic homeostasis system. This is confirmed by a number of experimental studies conducted by the authors[14,15], using highly sensitive radiometric equipment.
Temperature stability and electromagnetic homeostasis of the organism is preserved even in case of changes in environmental factors: temperature, pressure, man-made or cosmic radiation, magnetic and microwave fields[16]. The formation of the internal (endogenous) microwave field around the material of the dielectric implant poses the task of researching their radiative properties in order to determine the electromagnetic compatibility with the human body. The authors[14,15] conducted a study of some dielectric biomaterials for medical purposes. As a result, the radiation coefficient was determined and the presence of positive ("+") and negative ("-") microwave electromagnetic flows that can occur between the implant and living tissue was revealed. A significant influence of microwave flows on certain types of cells (oncological), at the local tissue level, and the whole human body was shown.
Currently, a significant number of materials for implantation have been developed and researched. They have both positive and negative properties. Most materials (especially synthetic ones) are characterized by the following features: the absence of a structure similar to bone tissue, the ability to withstand significant biomechanical loads, different levels of biophysical compatibility[17]. Since almost all of them are dielectrics, there is a need to study the electromagnetic properties of implantable bone substitute biomaterials before their use.
The scheme of the formation and interaction of EMR of a dielectric bone substitute with the human body tissues in a vertical section (projection) can be presented in the form shown in Figure 2. The area of bone (1) and soft tissue (2) of the human body, is in contact with the implant (3). The process of interaction of the installed implant (3) with the adjacent tissues (1, 2) with the formation of a positive ("+") electromagnetic flux is shown in Figure 2a, and a negative ("-") one is shown in Figure 2b.
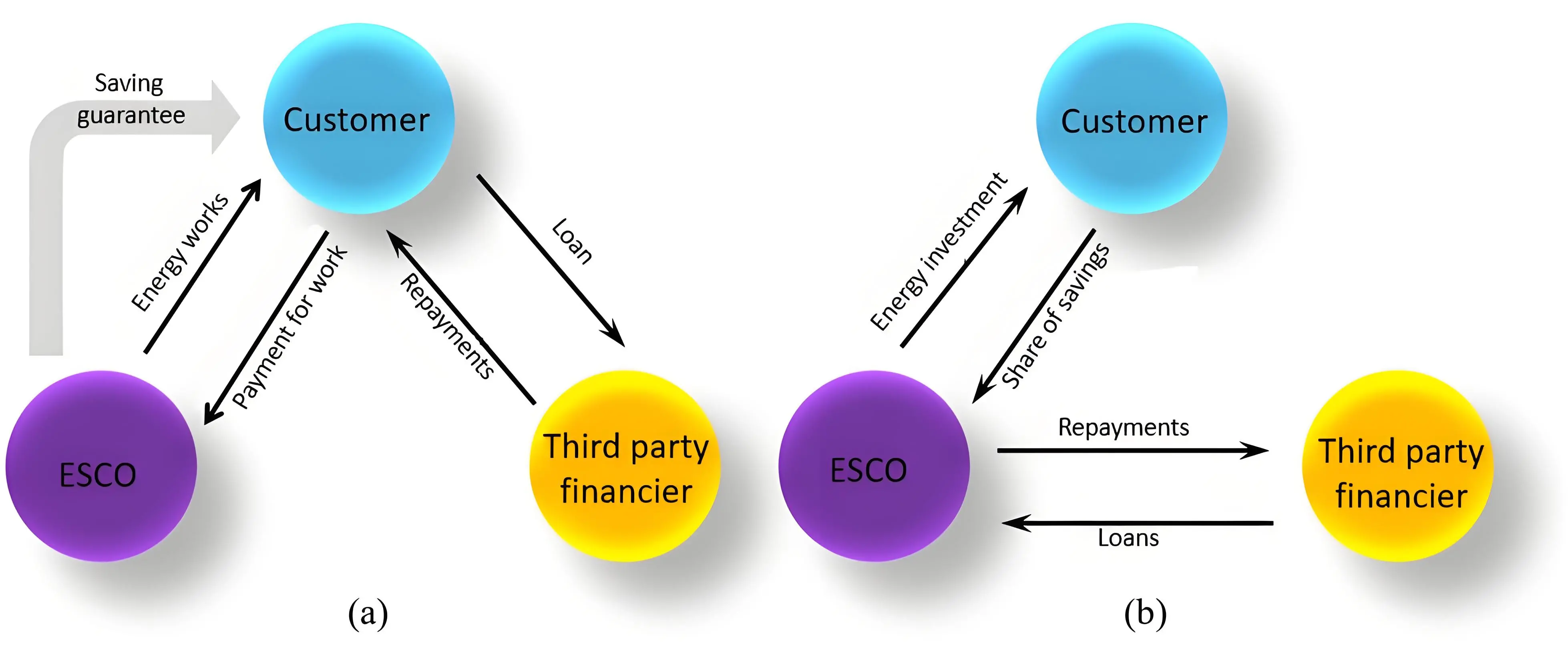
Figure 2. Schemes of formation of (a) positive ("+") flow and (b) negative ("-") flow of EMR. 1: bone tissue; 2: soft tissues; 3: implant; P1, P2: radiation power per unit area of bones and soft tissues of the body; P3: radiation power per unit area of the implanted material.
The powers of electromagnetic radiation (EMR) of the bone and soft adjacent tissues are naturally physiologically consistent with each other, and the level of radiation of the implanted bone-replaced material in the version of Figure 2a is higher than P1 and P2. In this way, the surrounding tissues receive constant additional radiation and a positive ("+") EMR flow is created in relation to the adjacent biotissue. In the version of Figure 2b, on the contrary, the implanted material absorbs EMR from the surrounding tissues, since its radiation level is lower and a negative ("-") EMR flow is formed.
Figure 3 shows three possible options for the interaction of the implantant (material) with the adjacent tissues of the human body in a horizontal section.
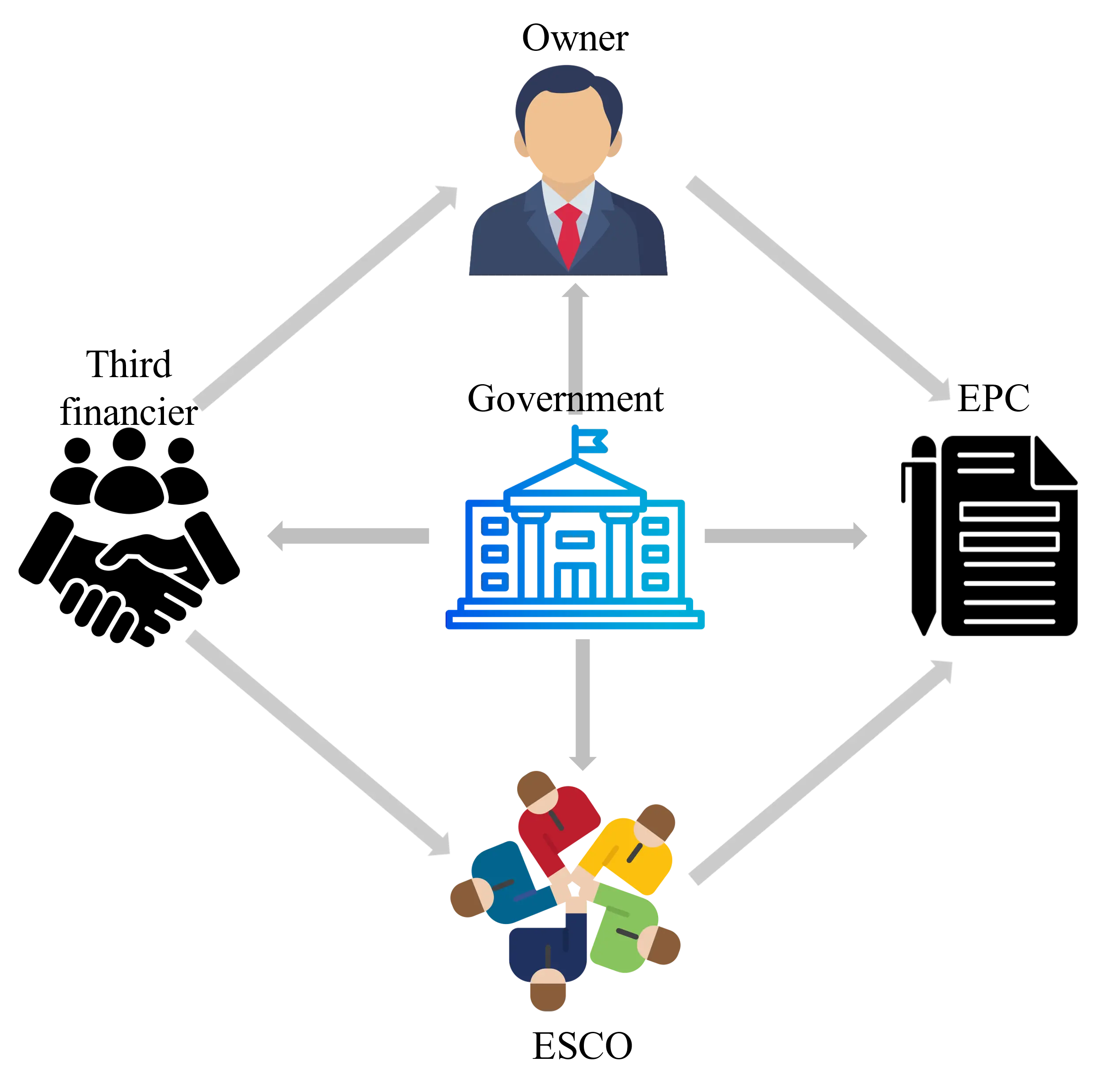
Figure 3. Scheme of interaction of microwave electromagnetic flows of the implant with adjacent tissues of the human body. 1: implantat; 2: adjacent tissues; T1, T2: temperature of the implantat and adjacent tissues; β1, β2: coefficients of the radiative capacity of the implantat and adjacent biotissue.
A dielectric implant 1 of natural or synthetic origin is surrounded by adjacent biotissues 2. The thermal field of the human body evenly heats both the implant and the adjacent biotissues, forming microwave fields that interact with each other.At the same temperature of these two objects, the electromagnetic interaction is determined by the radiation coefficient of the implant and biotissue. In these conditions, there are three options for the formation of such interaction, indicated in Figure 3. In the first option (Figure 3a), at the same temperature of the implant and biotissue (T1 = T2), as well as equal radiation coefficients (β1 = β2), an equilibrium interaction is established between them.This option is characterized by their full electromagnetic compatibility.In the second option (Figure 3b) at the same temperature (T1 = T2), the radiation capacity of the implantat is lower than that of the adjacent tissues (β1 < β2). The gradient of the distribution of the electromagnetic field is directed from the biotissue to the implant. This state is characterized by energy withdrawal from the implantat and the formation of a negative ("-") EMR flow in relation to the adjacent biotissue. A third option is possible (Figure 3c), when (T1= T2), but the radiation capacity of the implant is greater than that of biotissues (β1 > β2). In this case, the EMR flow is directed from the implant to the biotissue, characterized by the addition of energy and the formation of a positive ("+") flow. It should be noted that energy exchange in the form of microwave negative ("-") and positive ("+") flows between the implant and adjacent biotissues in the long term can change the course of reparative processes at the location of the implant and affect the effectiveness of clinical intervention. The power of the electromagnetic flow emitted by a physical body is determined by its temperature, and the level, in general, is described by the well-known law and Planck's formula. A low-intensity signal is formed at a human body temperature of 310 K, so its measurement is carried out using special highly sensitive equipment - a radiometric system (RS). The integrated EMF power (
where: k - Boltzmann's constant, 1.38 · 10-23 J/K ; T - thermodynamic temperature of the object;
For completely black body (CBB) βcbb = 1, at a temperature equivalent to the human body 310 K, and the bandwidth of the radiometric system on which the experimental studies were conducted 108 Hz, the value of the integral power, calculated by the formula (1)
Where βo - coefficient of radiation capacity of the implant or the human body. From formula (2), we get the expression for calculating the coefficients of the emissivity of the research objects
Thus, as follows from formula (3), in order to determine the coefficients of the emissivity of the implantat and the human body, it is necessary to measure the integral power of these objects of research and carry out appropriate calculations.
4. Technical Support and Research Technologies
The level of body radiation at a human temperature of 310 K in the microwave range is extremely low.Its measurement and registration requires specialized highly sensitive equipment—a radiometric system (RS).The authors of this study developed a non-standardized radiometric system for the frequency range of 37-53 GHz, which is and certified by the State Standard of Ukraine. The measurement band of the integral power, which is determined by the radiometric channel, is 108 Hz. The sensitivity approved by the RS certification is at the level of 3 · 10-14 W, which allows you to reliably measure the radiation of the human body, which is in the range (2.5-4.0) · 10-13 W. The experimental stand for measuring the radiation capacity of the human body and implant materials is located on the basis of the Department of Applied Radioelectronics of NTUU "KPI named after Igor Sikorsky". The stand includes a highly sensitive NU-2 (Hy-2) radiometric system and a standard thermostat TS-80M-2 (TC-80M-2) for heating materials up to human body temperature of 310 K, as well as a reference power generator for periodic checking of the RS. Research was conducted at a frequency of 51 GHz. The general method of conducting experimental studies and a detailed description of the procedure for measuring the emissivity of various biomaterials in order to determine their compatibility are given by the authors in[14]. To compare the radiation of the implant materials, at the beginning of each study, the average level of microwave radiation flowpower of the three respondents was evaluated.
5. Description of Research Objects and Assessment of Their Microwave Radiation Level (Experimental Part)
5.1 Research of materials for reconstructive and restorative surgery of facial bones
According to the agreement on scientific and technical cooperation between the National Technical University of Ukraine "Igor Sikorskyi Kyiv Polytechnic Institute" (department of applied radioelectronics) and the Bogomolets National Medical University (Department of Surgical Stomatology and Maxillofacial Surgery), implant materials and materials used in implantation for reconstructive and restorative surgery of facial bones were investigated.
The investigated biomaterials were divided into two main groups by origin.
Natural origin:
1. Human body—measurement of the EMR flow from the middle of the inner side of the palm;
2. Whole vertebra—a whole tubular bone of animal origin;
3. Fine bone tissue of a vertebra—a tubular bone of animal origin;
4. Cerabone, BotissDental—a bone substitute material of animal origin (granules 0.5-1.0 mm), which has spatial stability, hydrophilicity of the surface of its particles, which retains similarity with human bone (surface, porosity, chemical composition). The term of its resorption 6-9 months[13,18];
5. Parasorb (Resorba)—collagen cones made of native collagen fibers of the horse, which provide fast and reliable hemostasis, stabilization of thrombus in bone defects and further tissue regeneration within 2-4 weeks;
6. Gelatamp (Roeko)—a porous hemostatic sponge, which includes hardened gelatin (9.5 mg) and colloidal silver (0.5 mg), with a absorption time of up to 4 weeks.
Synthetic origin:
7. Medical glue with folic acid (MG + FA);
8. Medical glue without folic acid (MG-FA) is a long-acting biocomposite based on reticulated polyurethane, which has the ability to polymerize in the bone cavity, is used as a barrier membrane, and gradually dissolve within 9-12 months[12,17];
9. Hydroxyapatite powder (HA)—the material is synthesized from an aqueous solution of calcium and phosphorus salts, which can be used in combination with other components of multiphase ceramics or cover the surface of implants;
10. Syntekist-glass-ceramic powder (300-500 microns), used to fill bone defects, as well as to influence the process of reparative osteogenesis;
11. Biomin GT 700—preparations of two-phase ceramics based on hydroxyapatite and tricalcium phosphate with particles of different sizes;
12. Biomin GT-500 (powder).
The complete list of research objects by origin is given in Table 1.
Research objects | |||
Object number | Natural origin | Object number | Synthetic origin |
1 | Human palm (average level of radiation) | 7 | MG + FA |
2 | Complete vertebra | 8 | MG-FA |
3 | Crushed bone tissue of the vertebra | 9 | Hydroxyapatite (powder) |
4 | Cerabon (granules) | 10 | Syntekist (powder) |
5 | Collagen sponge | 11 | Biomin GT-700 (powder) |
6 | Gelatin sponge | 12 | Biomin GT-500 (powder) |
MG: medical glue; FA: folic acid.
The results of experimental measurements of the integral power and calculations of the coefficients of the radiative capacity of the studied objects β1 are given in the Table 2.
Research objects | Power P∑; | Coefficient β1 | |
P∑, 10-13 W | β1 = P∑/PCBB ; | % from β2 | |
1 | 2.3 | 0.54 | 100 |
2 | 1.8 | 0.42 | 77 |
3 | 1.1 | 0.26 | 47 |
4 | 0.6 | 0.14 | 26 |
5 | 0.2 | 0.05 | 8 |
6 | 0.2 | 0.05 | 8 |
7 | 0.6 | 0.14 | 24 |
8 | 0.4 | 0.09 | 18 |
9 | 0.2 | 0.05 | 8 |
10 | 0.1 | 0.02 | 4 |
11 | 0.1 | 0.02 | 4 |
12 | 0.1 | 0.02 | 4 |
Table 2 covers the numbering of the investigated implant materials; integrated power measured by a radiometric system with an analysis band of 100 MHz at 51 GHz; the value of the emissivity of each material, calculated as the ratio of the measured integral power to the calculated integral power of a completely black body for a human body temperature of 310 K and the percentage ratio of the material's integral power to the integral power of the human body.
Figure 4 shows a diagram of the distribution of the studied materials depending on the value of the spectral density of the radiation power per unit area of the implant material and the percentage of deviation (mismatch) from the radiation level of the human body. As a result of the conducted experimental studies, three groups of materials were distinguished according to the level of electromagnetic compatibility.
Analysis of the results of experimental studies of the radiative capacity of materials for implants given in the Table 2 and Figure 4, shows a significant distribution of data, both in terms of the radiation power level and its coefficient. It should be noted that the radiation coefficient of implant materials of natural origin (researchobjects 2-4) is close to the radiation capacity of the human body and has a difference (less) of 1, 3-5 times.The use of these materials creates weak negative effects of EMR, so they can be considered electromagnetically compatible with the human body.
Some of the materials (researchobjects 7-9) have up to 15 times lower emissivity, and accordingly generate a significant negative EMR flux, so they can be classified as insufficiently compatible. The last group (researchobjects5, 6, 10-12) is characterized by a significantly reduced level of radiation (15-25 times less), the formation of negative EMR flows from the tissues in the direction of the specified materials, which are considered energetically incompatible and unfavorable for long-term contact with the tissues of a living organism, especially with large volumes of their use.
Figure 5 shows the conditional distribution of experimentally studied implant materials regarding their electromagnetic compatibility with the human body.
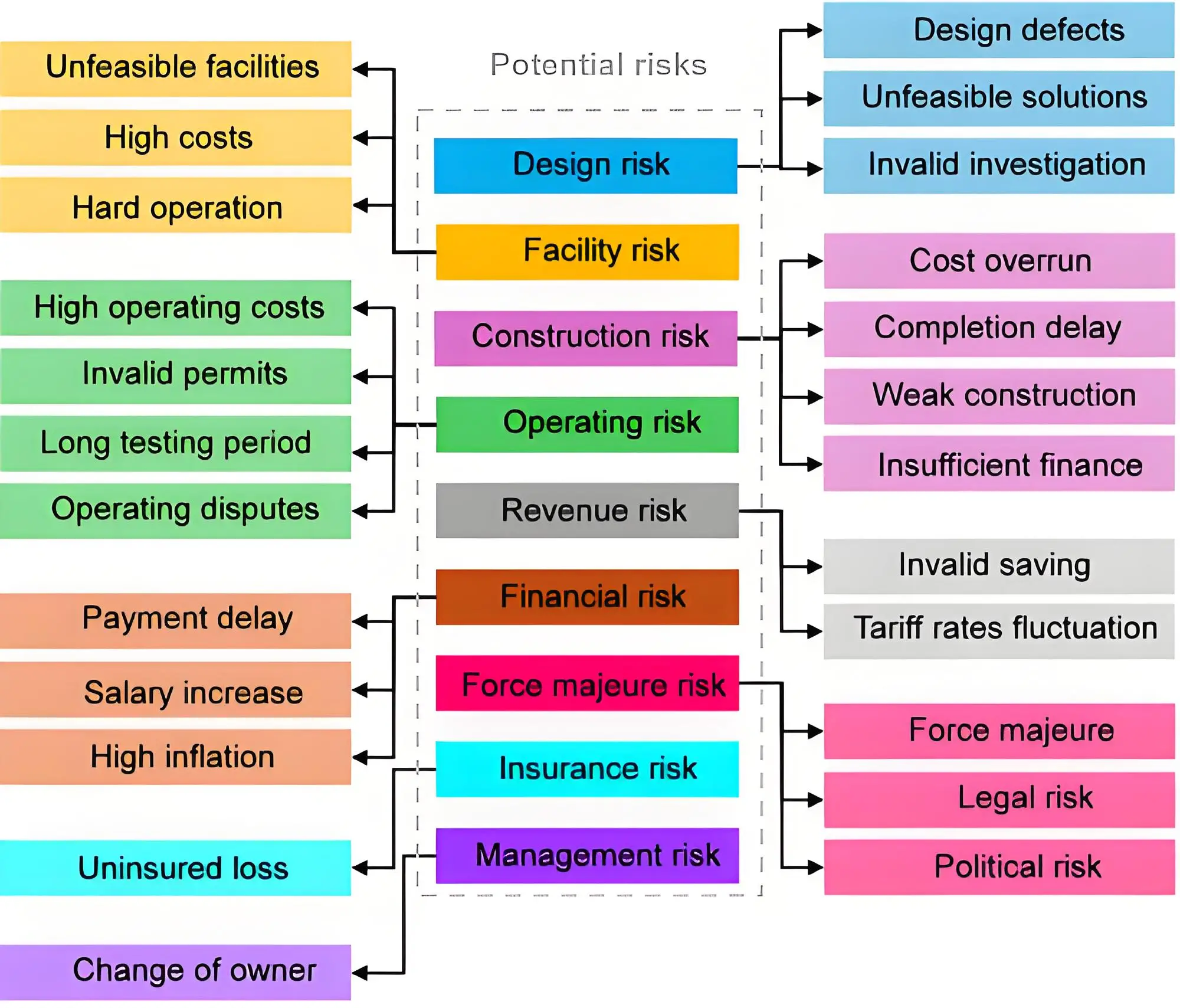
Figure 5. Groups of research objects according to electromagnetic compatibility with the human body.
The first group includes implant materials that have an electromagnetic emission power 1.3-5 times less than that of the human body and which can be considered compatible; the second includes materials that emit electromagnetic waves in the millimeter range with a power 5-15 times lower than the radiation of the human body and which can be considered conditionally compatible; the third group includes materials whose power of electromagnetic radiation in the millimeter range is 15-25 times less than the electromagnetic radiation of the human body, and which are poorly compatible with living tissues of the human body.
5.2 Microwave electromagnetic assessment of dental filling materials
The authors conducted experimental studies of the radiation capacity of dental filling materials and its evaluation[18,19]. For this, the radiometric system described in the previous section was used and the following dental materials were selected[20,21].
Sample No. 1—material based on the resorcin-formalin mixture "Foredent" (Spofa, Slovakia) has high antimicrobial properties, but has a number of shortcomings that have led to its rejection in many countries: cytotoxic, stains tooth hard tissues and even surrounding soft tissues, is not sufficiently adhesive.
Sample No. 2—glass-ionomercement "Endion" (Voco, Cuxhaven, Germany) consists of fine fluoroalumosilicate glass and polycarboxylic acid. It has a unique ability to chemical connection with dentine and ensure reliable tightness of the root canal, but is not stable enough under the action of mechanical loads. It's difficult to remove it from the root canal if retreatment is necessary. Allthose caused its unpopularity for the clinic applications.
Sample No. 3—Zinc oxide-eugenol material "Endomethazone" (Septodont, Paris, France) has an antiseptic effect due to eugenol, but the presence of a certain amount of free eugenol causes an inflammatory tissue reaction. This property they try to balance by the introduction of steroid preparations into the material, which is undesirable, since general resorptive effects are possible.
Sample No. 4—polymeric cement "AH Plus" (Dentsply, Charlotte, NC, USA)—epoxy-amine polymer, cures by polyaddition reaction after mixing two pastes. A very plastic material of uniform consistency, forming a thin film, but, however, gives a fairly large curing shrinkage. It has some cytotoxicity during the curing process, which causes an inflammatory response, but the hardened material is tolerant to the surrounding tissues, and reactive inflammation quickly passes.
Sample No. 5—light-cured composite "Spectrum" (Dentsply, Charlotte, NC, USA) (color A3.5)—is a radiopaque photopolymerizable universal hybrid composite with an ultra-fine particle size for the restorations of the frontal teeth and molars. Barium glass and sintered silicon with an average particle size of 0.8 microns are used as fillers. It has excellent mechanical and aesthetic properties.
Sample No. 6—chemical curing composite "Compolux" (Septodont, Paris, France) based on Bis-GMA resin; the filler has a particle size of up to 20 microns and is 63% by weight. It is quite mechanically stable, and slightly aesthetic.
Sample No. 7—glass-ionomer cement "Cavitan-plus" (Spofa, Slovakia). In addition to the above ability to form a chemical bond with hard tooth tissues, common to glass-ionomers, there is another remarkable property—to excrete fluorine, which is the basis of anti-caries action. This cement is used for linings, temporary (long-term) and permanent fillings in permanent and decidual teeth.
Sample No. 8—natural tooth enamel.
Sample No. 9—natural tooth dentine.
Sample No, 10—spongy bone cut.
Patterns with a square of 0.5 cm2 and a thickness of 1 mm, with the possibility of overlapping the cross section of a standard waveguide in the frequency range of 37-53 GHz, were prepared from these materials.
In the course of the experiment, the radiation intensity of each material was checked at a temperature of 37 °C, the level of which was recorded by a measuring unit NU-2 with a sensitivity of 10-14 W at a frequency of 52 GHz. According to our measurements, the radiation power of the considered range of dental materials was concentrated in the range (1.8-3.1) · 10-13 W/cm2. Taking formulas (1, 2) into account, the gray factor (factor ) of the material was calculated. The results are presented in the nomogram (Figure 2).
Samples No. 1, 2, 5 and 7 are used to fill the root canals of the teeth, therefore, the properties under study should be compared with the similar properties of dentin (No. 8) with which they come in contact. Samples of materials No. 3, 4, 6 are used to seal the tooth surface; therefore, their properties should be compared with the properties of tooth enamel (No. 9).
Thus, the identification of the grayness coefficient (ß coefficient) deviates up to 38.0% when comparing some materials No. 1,2,5,7 with dentine material No. 8. The materials used to restore the surfaces of teeth No. 3, 4, 6 have a smaller spread from 0% to 10.8%, compared to tooth enamel material No. 9.
The method of microwave electromagnetic identification (compatibility assessment) of dental materials is also promising for use in the development of new dental materials.
6. Analysis and Conclusions of the Conducted Research
6.1 Preliminary analysis of research results
The authors performed theoretical and experimental studies, which consist of the following:
- A review of the physical foundations of the emergence and formation of microwave electromagnetic fields and radiation at the implant-adjacent biotissue interface;
- Determined coefficients of radiation capacity of known and widely used contactinf materials in dentistry;
- A comparison of their radiative capacity with a similar parameter of biotissues of the human body was made;
- In the microwave range, instrumental experimental studies and measurements of electromagnetic radiation of a number of contacting dental materials were performed;
- A percentage assessment of their electromagnetic compatibility with the biotissue of the human body was carried out.
Microwave electromagnetic radiation of a dielectric implantation material or an implant from it is a constant value determined by its composition and the temperature of the environment, which in this case is the temperature of the human body and is ≈ 310K. The difference in radiation coefficients of human body tissue and the implanted material, despite the constancy of the temperature at the point of contact, can lead to the occurrence of positive ("+") or negative ("-") flows of electromagnetic energy and the occurrence of electromagnetic incompatibility. With large gradients (differences) of EMR flows (more than 50%) and their long-term influence during the use of the implant, or the prolonged effect of the smaller difference of these flows affects the processes of tissue regeneration and increases the risks of developing postoperative complications, especially with significant volumes of implanted substances. Considering the results of experimental studies of radiation ability, it is advisable to divide implant materials for reconstructive and restorative operations on facial bones into several groups according to electromagnetic compatibility, as shown in Figure 5. Materials of natural origin are more favorable, and materials of synthetic origin from inorganic mineral substances are less favorable.
6.2 Final conclusions
1. The data presented in the tables indicate that the intensity levels of electromagnetic radiation of the implantation materials can differ significantly from each other.
2. If the level of electromagnetic radiation of the implant is greater than the radiation of the surrounding biological tissues, then a positive flux is formed, which is absorbed by the biological tissues, and if it is less, then on the contrary, the implant absorbs the radiation of the biological tissues.
3. Materials of the II and III groups (Figure 5) under the condition of long-term contact can contribute to the deterioration of the process of reparative regeneration of tissues due to their energy suppression or exhaustion.
4. The usage for the regeneration of bone tissue materials compatible with microwave electromagnetic radiation will allow maintaining the optimal level of reparative processes, contribute to the physiological or increased efficiency and predictability of the results of surgical interventions, shorten the healing period and better restore the structural and functional state of tissues.
5. Studies of electromagnetic compatibility of materials and tissues of the human body require further study, both in laboratory conditions and in clinical practice.
Acknowledgement
Gratitude—to the Radiotechnical Faculty of the Kyiv Polytechnic Institute named after Igor Sikorsky for supporting research in this area.
Author contributions
All authors contributed equally to this work.
Conflicts of interest
All authors declare that they are bound by confidentiality agreements preventing disclosure of conflicts of interest in this work.
Ethical approval
Not applicable.
Consent to participate
Not applicable.
Consent forpublication
Not applicable.
Availability of data and materials
None
Funding
None.
Copyright
© The Author(s) 2024
References
-
1. Safavi MS, Khalil-Allafi J, Ahadzadeh I, Walsh FC, Visai L. Improved corrosion protection of a NiTi implant by an electrodeposited HAp-Nb2O5 composite layer. Surf Coat Technol. 2023;470:129822.
[DOI] -
2. Safavi MS, Khalil-Allafi J, Restivo E, Ghalandarzadeh A, Hosseini M, Dacarro G, et al. Enhanced in vitro immersion behavior and antibacterial activity of NiTi orthopedic biomaterial by HAp-Nb2O5 composite deposits. Sci Rep. 2023;13(1):16045.
[DOI] -
3. Safavi MS, Khalil-Allafi J, Visai L. Improved osteogenic activity of NiTi orthopedic implant by HAp-Nb2O5 composite coatings: Materials and biological points of view. Biomater Adv. 2023;150:213435.
[DOI] -
4. Pratap B, Gupta RK, Bhardwaj B, Nag M. Resin based restorative dental materials: Characteristics and future perspectives. Jpn Dent Sci Rev. 2019;55(1):126-138.
[DOI] -
5. Yanenko O. Low-Intensive Microwave Signals in Biology and Medicine. J HumPhysiol. 2019;1(1):29-41.
[DOI] -
6. Pyuryk VP, Prots GB, Ogienko SA, Pyuryk YV. Combined use of autologous bone marrow and artificial bone substitutes to replace postoperative bone defects. Visn Pro Bio Med. 2014;2:105-109.
-
7. Zhao R, Yang R, Cooper PR, KhurshidZ , Shavandi A, Ratnayake J. Bone grafts and substitutes in dentistry: A review of current trends and developments. Molecules. 2021;26(10):3007.
[DOI] -
8. Cordonnier T, Sohier J, Rosset P, Layrolle P. Biomimetic materials for bone tissue engineering-state of the art and future trends. Adv Eng Mater. 2011;13(5):B135-B150.
[DOI] -
9. Tour G. Craniofacial bone tissue engineering with biomimetic constructs [dissertation]. Sweden: Karolinska Institutet; 2012. Available from: https://www.proquest.com/openview/56ab
-
10. Zhu W, Nie X, Tao Q, Yao H, Wang DA. Interactions at engineered graft-tissue interfaces: A review. APL Bioeng. 2020;4(3):031502.
[DOI] -
11. Yanenko O, Shevchenko K, Peregudov S, Malanchuk V. Low Intensity Microwave Fields and Radiation and Their Interaction with the Human Body. J Hum Physiol. 2021;3(2):40-50.
[DOI] -
12. Sculean A, Nikolidakis D, Schwarz F. Regeneration of periodontal tissues: combinations of barrier membranes and grafting materials - biological foundation and preclinical evidence: a systematic review. J Clin Periodontol. 2008;35(8 Suppl):106-116.
[DOI] -
13. Gozhenko A, Gorbachevsky OV. Electromagnetic homeostasis and human adaptation to stress-factors. Visn Nac Akad Pedagog Nauk Ukr. 2009;10:12-21. Ukrainian. Available from: http://dspace.nbuv.gov.ua/handle/123456789/26491
-
14. Yanenko O, Peregudov S, Shevchenko K, MalanchukV, GolovchanskaO. Assessment of dielectric implantable biomaterials compatibility based on the level of low-intensity mm-range signals. In: 2020 IEEE 40th International Conference on Electronics and Nanotechnology (ELNANO); 2020 Apr 22-24; Kyiv, Ukraine. New York: IEEE. 2020. p. 436-441.
-
15. Yanenko O, Shevchenko K, Malanchuk V, Golovchanska O. Microwave Evaluation of Electromagnetic Compatibility of Dielectric Remedial and Therapeutic Materialswith Human Body. Int J Mater Res USA. 2019;7(1):37-43.
[DOI] -
16. Piszczek P, Wojcik-Piotrowicz K, Gil K, Kaszuba-Zwoinska J. Immunity and electromagnetic fields. EnvironRes. 2021;200 Suppl 2:111505.
[DOI] -
17. Yoshikawa H, Myoui A. Bone tissue engineering with porous hydroxyapatite ceramics. Int J Artif Organs. 2005;8(3):131-136.
[DOI] -
18. Yanenko OP, Peregudov SM, Golovchanska OD, inventor. Method for measuring electromagnetic signal power and identifying dental materials. Ukrain patent NO49357. 2008.
-
19. Krasiuk OD, Golovchanska OD, Peregudov SM, Yanenko OP. Compatibility assessment of dentalmaterials. Bulletin of NTUU "KPI": Series instrument manufacturing. 2008;35:143-147. Ukrainian. Available from: https://ela.kpi.ua/server/api/core/bitstreams/cd027549-0bd8-41a0-a027-7ca9beae56d9/content
-
20. van Nurt R. Fundamentals of Dental Materials Science. 2nd ed. Edinburgh: Mosby; 2002.
-
21. Danilevskyi NF, Borysenko B, Sidelnikova LF, Nesin AF. Therapeutic dentistry: Tom 1. Kyiv: Medicina. 2017. Ukrainian.
Copyright
© The Author(s) 2023. This is an Open Access article licensed under a Creative Commons Attribution 4.0 International License (https://creativecommons.org/licenses/by/4.0/), which permits unrestricted use, sharing, adaptation, distribution and reproduction in any medium or format, for any purpose, even commercially, as long as you give appropriate credit to the original author(s) and the source, provide a link to the Creative Commons license, and indicate if changes were made.
Publisher’s Note
Share And Cite