Abstract
Biodegradable pH-responsive hydrogels have become a powerful tool in medicine for synthesizing wound dressings and drug delivery applications. The current study focuses on the development of crosslinked semi-interpenetrating polymer networks of chitosan/poly(ethylene glycol)/3-glycidyloxypropyltrimethoxy silane (GPTMS) blends containing honey and aloe vera gel. All hydrogels were prepared by physical blending and solution casting methods. Fourier transform infrared (FTIR) spectroscopy was performed to analyse the developed interactions among the polymers and crosslinker. To check the thermal behaviour and crosslinking density of all hydrogel matrixes, thermogravimetric analysis (TGA) was done. The effects of the crosslinker concentration on the swelling trend of all hydrogels were investigated against distilled water and buffers of varying pH. The hydrogel was found thermally stable with enhanced swelling rate, good moisture capability, and pH responsiveness. These properties and the material's cost-effectiveness suggest that the fabricated blend hydrogels can be suitable for wound dressing and other biomedical applications.
Keywords
1. Introduction
The wound-healing phenomenon comprises complicated biochemical processes at the cellular level, which can be accelerated with the help of suitable wound dressings[1,2]. The conventional wound dressing is dry-like pledget, which may not have a better effect as the eschar can be formed on renewed skin during healing[3,4]. This problem can be removed using wet wound dressings such as hydrogel, made of low-cost material with good biocompatibility, water retention capacity, absorption, and ventilating ability. The hydrogel-based wound dressing can be applied and removed easily without causing any injury to the tissues[5-7]. Hydrogels are three-dimensional semi-interpenetrating polymer networks of natural or synthetic polymers with good swelling properties[8-10]. Currently, numerous wound dressing polymers are available, including chitosan(CS), gelatine, polyethylene glycol (PEG), polyvinyl pyrrolidone, and xanthan gum[11,12]. Chitosan is comprised of a repeating monomer unit known as N-acetyl-D-glucosamine, linked through (1-4)-linkages to form elongated chains. Within the monomer structure are functional groups: primary amines, hydroxyl groups, and secondary hydroxyl groups (OH)[10]. These functional groups provide sites for potential chemical modifications without altering the degree of polymerization. These reactive groups within the chitosan backbone allow for convenient customization, either through physical or chemical means, facilitating the fabrication of scaffolds with tailored properties suitable for various healthcare and other biomedical applications[13]. It has been used in the composition of drug delivery systems and wound dressings due to low toxicity, biodegradability, anti-microbial properties, biocompatibility, and formation of epidermal scaffolds[12,14-17].
Chitosan-based hydrogels exhibit a great degree of swelling and show changes in swelling behaviour when they come in contact with any stimulus, such as pH[18,19]. These hydrogels can induce the deposition of regular collagen, proliferation of fibroblasts, and synthesis of hyaluronic acid at wound sites, leading to faster healing and prevention of scars[20,21]. Chitosan-based fully swollen hydrogels have low interfacial tension between body cells and hydrogel surface, which can reduce cell adhesion and absorption process[22]. A synthetic polymer like PEG can be blended with chitosan to improve the properties of hydrogel[23,24].
PEG is a hydrophilic and biocompatible ethylene oxide polymer formed by the polymerization of ethylene glycol oligomers or when ethylene oxide reacts with ethylene glycol[25]. It is a synthetic polymer composed of repeating ethylene oxide units with a molecular weight of 44 Daltons[26]. PEG has been proven to be an effective material for biomedical applications, including the formation of drug and cell delivery vehicles and the promotion of tissue regeneration, as it does not show any immune response[27-29]. PEG-based hydrogels can direct cell proliferation, secretion, and differentiation[30,31].
CS and PEG blends can be crosslinked using various silane-based coupling agents like aminopropyl triethoxysilane, 3-Glycidyloxypropyltrimethoxy silane (GPTMS), and methoxy-terminated polydimethyl siloxane to increase the durability of hydrogel[32]. The GPTMS is of paramount importance due to its multi-functionality. It belongs to the family of organo-silanes, which has an epoxy group and three methoxy groups at opposite terminals of the chain. Both epoxy and methoxy rings are reactive: epoxy towards acids, alcohols, thiols, and amides, and methoxy towards glass[33-35]. Li et al. fabricated self-healing injectable hydrogels composed of chitosan, PEG, and octa-functionalized crosslinker for wound healing application. The resulting hydrogels had great mechanical strength, reasonable cytocompatibility, and high healing efficiency. Cell proliferation and migration acceleration were observed in diabetic mice with minimum inflammatory cells using these hydrogels[43]. Chen et al. reported the development of chitosan/PEG-based hydrogels with improved mechanical properties and suitable degradation rates for wound healing applications. These hydrogels increased the fibroblast proliferation rate and supported epithelial migration[44]. In another study, Hasan et al. prepared CS-PEG-based hydrogels and evaluated them in rat skin wound models[45].
Traditionally, aloe vera, with medicinal properties, has been utilized to address skin ailments like burns, wounds, and inflammation. Additionally, aloe vera exhibits a range of therapeutic attributes, including anti-cancer, antioxidant, anti-diabetic, and anti-hyperlipidemic properties. It comprises over 75 diverse compounds, encompassing vitamins (such as A, C, E, and B12), enzymes (like amylase, catalase, and peroxidase), minerals (including zinc, copper, selenium, and calcium), sugars (e.g., monosaccharides like mannose-6-phosphate and polysaccharides such as glucomannans), anthraquinones (such as aloin and emodin), fatty acids (like lupeol and campesterol), hormones (such as auxins and gibberellins), and other constituents (including salicylic acid, lignin, and saponins)[40,41]. Honey, on the other hand, a natural substance rich in sugars, serves as a food sweetener, whole food, and medicinal aid. Epidemiological research has highlighted its potential protective and therapeutic effects on health, enhancing immune function, antibacterial properties, and antioxidant responses, with potential benefits extending to cardiovascular health. Comprising primarily glucose and fructose, honey contains over 180 additional components, such as proteins, amino acids, essential minerals, vitamins, enzymes, and phenolic phytochemicals[42]. Honey and aloe vera can be added to the hydrogel to enhance its antimicrobial and antioxidant potential for wound healing. Aloe vera is reported to increase the drug absorption level, while honey can enhance the antimicrobial effect[36-39].
In this study, we have synthesized a range of novel hydrogels for future biomedical applications using chitosan, polyethylene glycol, honey, and aloe vera. The incorporation of GPTMS crosslinker into these hydrogels has been meticulously executed to crosslink the polymer material. The prepared hydrogels were analysed using FTIR and TGA, while the important swelling studies were performed against distilled water, buffer solutions of varying pH to elucidate the pH responsiveness of these hydrogels. This work has not been reported previously.
2. Experimental Section
2.1 Chemicals
CS was bought from Germany with 406,039 g/mol with 75% DDA. PEG (600 g/mol polyethylene glycol), CH3OH (absolute methanol), CH3COOH (100 % pure acetic acid), HCl (37% pure hydrochloric acid), and HCOOH (95% pure formic acid) were purchased from Merck, Germany. NaOH (98.0% pure sodium hydroxide), GPTMS (silane-based crosslinker), CH3COONa (99.995% pure sodium acetate), KH2PO4 (99% pure potassium dihydrogen phosphate), and KCl (99% pure potassium chloride) were obtained from Sigma Aldrich [Milwaukee, WI]. All chemicals were pure and brought from Sigma Aldrich and of analytical grade, while aloe vera and honey were of food grade. Aloe vera and honey were bought from a local vendor. All chemicals were used without any processing.
2.2 Fabrication of hydrogel
All hydrogels were prepared by physical blending and solution casting methods[46,47]. A PEG solution was prepared by dissolving 0.1 g of PEG in 15 mL of distilled water and stirring for 1 hour at 60 °C. A CS solution was formed by adding 1 g of chitosan to 70 mL of 2% formic acid and stirring at 60 °C until the polymer was completely dissolved. The PEG and CS solutions were mixed and blended for an additional hour at the same temperature. To crosslink the hydrogel, 100 μl of GPTMS was mixed with 10 mL of methanol and then added dropwise into the above blend. This blend was named as PCG (control sample).
For honey-based hydrogels, 10 mL of 0.5% and 1% honey solutions (in distilled water) were blended with the PEG-CS mixture by stirring for 2 hours. To crosslink the hydrogel, 100 mL of GPTMS was mixed with 10 mL of methanol and then added dropwise into the above blend. The solution was stirred for an additional hour at 60 °C to ensure complete crosslinking. The honey-containing gels were labelled HPCG1 and HPCG2, respectively (Table 1). A similar procedure was followed for aloe vera-based hydrogels by varying the concentration to 1 g and 0.5 g, labelled APCG1 and APCG2, respectively.
Sample | CS (g) | PEG (g) | Crosslinker (μL) | Honey (%) | Aloe vera (g) |
PCG | 1 | 0.1 | 100 | - | - |
APCG1 | 1 | 0.1 | 100 | - | 1 |
APCG2 | 1 | 0.1 | 100 | - | 0.5 |
HPCG1 | 1 | 0.1 | 100 | 0.5 | - |
HPCG2 | 1 | 0.1 | 100 | 1 | - |
CS: chitosan; PEG: polyethylene glycol.
All prepared polymeric blends were cast into petri dishes and dried at 55 °C. The resulting hydrogel films were then peeled off and stored in a moisture-free environment for further use.
2.3 FTIR characterization
The hydrogel FTIR spectra were obtained using Bruker Alph-P FTIR Spectrophotometer with transmittance mode to characterize the presence of functional groups of incorporated material and their possible cross-linking. The spectra were recorded in the range of 4,000-480 cm-1 wavenumber in 64 scans with 2 cm-1 resolution. The average of all scans for each sample was taken for analysis, and the spectra were normalized.
2.4 TGA analysis
Thermogravimetric examination of all crosslinked polymer networks was done using SDT build 95 module DSC-TGA standard. The nitrogen flow rate was maintained at 15 mL per minute. The temperature increment rate was set as 20 °C per minute from room temperature to 600 °C.
2.5 Swelling studies against distilled water and varying pH
The previously described procedure was followed to check the response of all hydrogels in distilled water and buffer solutions of varying pH[48]. All hydrogel samples were cut into round pieces weighing 30 mg each in dry form. These pieces were immersed in solvent (20 mL) in different containers. After equal intervals of 10 min, the solvent was removed, and the surplus amount was gently soaked out of hydrogel using tissue paper. The swollen pieces were weighed after every interval. To check hydrogel response to the change in pH, buffer solutions with varying pH (2, 4, 6, 7 and 8) were prepared by following the standard procedure using a pH meter (pH-870 model, Tecpel). A similar procedure was followed to check the swelling rate of all hydrogels against pH.
The process for each experiment was continued until there was no further change in the weight of the swollen hydrogel (equilibrium point). The experiment was performed thrice, and the mean value was determined. Using equation 2.1, the degree of swelling was calculated.
While,
WD = mass (g) of hydrogel in dry form.
Ws = mass (g) of the swollen hydrogel at a time interval 't'.
3. Results and Discussion
3.1 Structural analysis
FTIR study was performed to investigate the incorporation of chemical groups and possible crosslinking in the hydrogels. Figure 1 represents the spectral information of all hydrogel films. The appearance of broad band in the 3,509-3,116 cm-1 range indicates -OH stretching of intra- and inter-molecular hydrogen bonds in the prepared hydrogels[49]. A definite peak at 2,877 cm-1 can be noticed, associated with the stretching vibrations of -CH groups[50]. Chitosan incorporation is proved by the C=O stretching vibration and N-H bending vibration. C=O stretching vibration of amide I appeared at 1,649 cm-1 and is consistent in all hydrogels, while the N-H bending vibration of amide II has a characteristic peak at 1,576 cm-1 [51,52]. The stretching bands at wavenumbers 1,110 cm-1 and 1,230 cm-1 could be associated with the acyclic -C-O-C group (PEG) and cyclic -C-O-C group (CS)[53,54]. A peak was observed around 892 cm-1, which could be due to the pyranose ring of CS[55]. The incorporation of GPTMS can be confirmed via the vibrational activity of -Si-O-Si-, which showed absorption at 1,280 cm-1 and 1,070 cm-1 [55,56]. A peak at 1,028 cm-1 is noticed in all samples, confirming the covalent bond formation (Figure 2) between CS and PEG[53]. Moreover, a peak at 3,410 cm-1 indicates the hydrogen bond between NH2 of CS and OH of GPTMS[57].
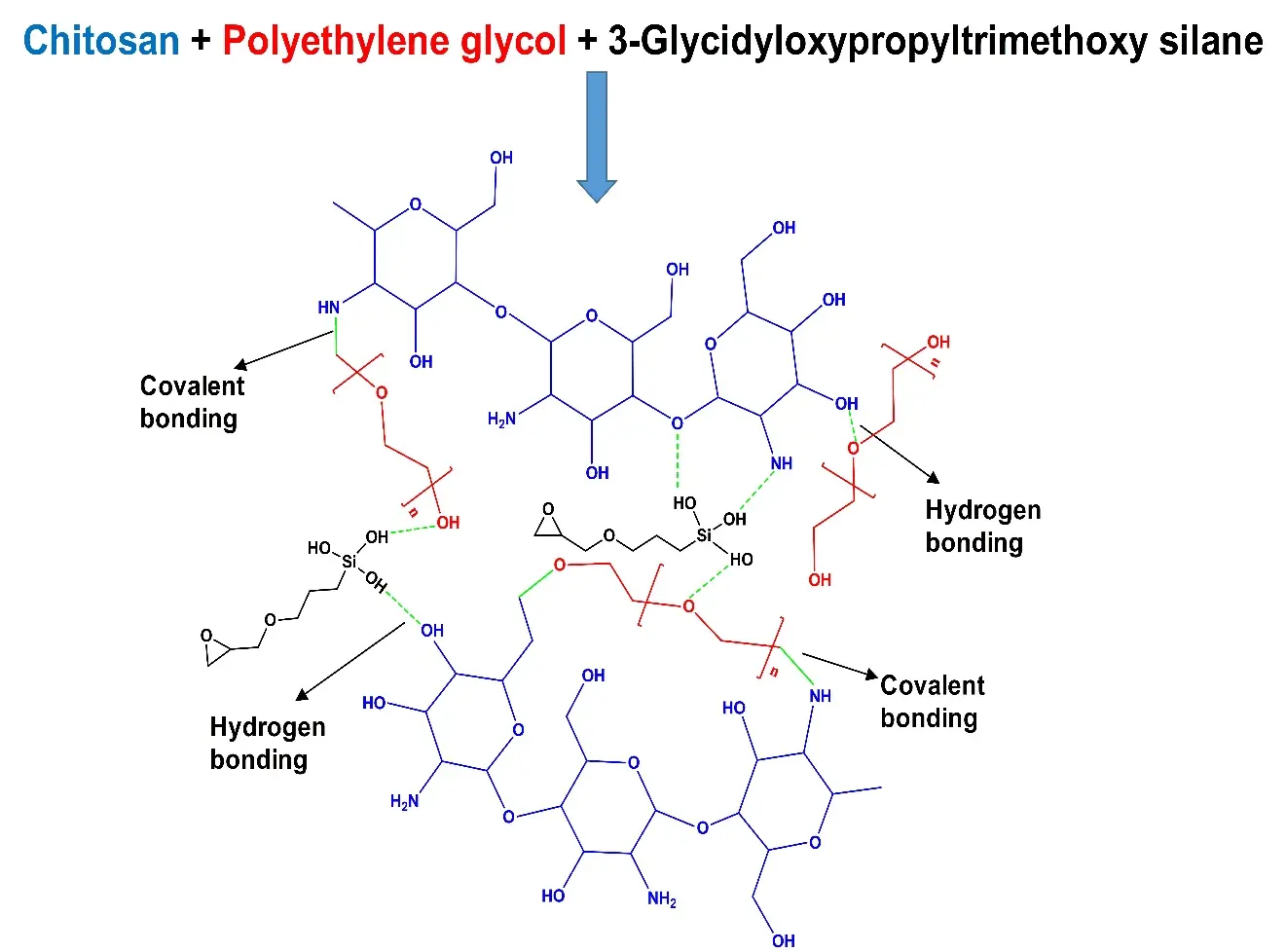
Figure 2. Proposed crosslinked CS-PEG-GPTMS hydrogel matrix. CS: chitosan; PEG:polyethylene glycol; GPTMS: 3-Glycidyloxypropyltrimethoxy silane.
A broad band of absorption at wavenumber 3,424 cm-1 and 1,743 cm-1 can be seen in all APCG hydrogels that could be referred to as stretching of hydroxyl and carbonyl groups, respectively. Meanwhile, peaks at 1,643 cm-1 and 1,418 cm-1 showed symmetric /asymmetric stretching of the uronic acid -COO present in aloe vera. The absorption at 1,256 cm-1 indicated the presence of acetyl -C-O-C- bonds, which are present in mannose sugar, confirming the integration of aloe vera gel[58].
In the FTIR spectra of honey-based hydrogels, prominent peaks are observed at 3,700 cm-1 and 3,000 cm-1, corresponding to stretch vibrations attributed to carbohydrates, water, and organic acids, respectively. The 2,900-2,800 cm-1 absorption band indicates C-H stretching vibrations within the sugar molecules. Additionally, a peak at 1,640 cm-1 signifies the presence of bending vibrations of OH and stretching vibrations of the ketone functional group in fructose and glucose, respectively. Carbohydrates exhibit stretching vibrations and bending of the C-H group in the fingerprint region from 1,450 to 700 cm-1, owing to their chemical skeleton comprising C-O, C-C, and C-H bonds[59,60].
3.2 TGA analysis
TGA analysis was performed to investigate the temperature-dependent weight loss profile and the influence of varying concentrations of incorporated ingredients on the thermal stability of fabricated crosslinked hydrogels. The data was plotted between % weight loss (at the Y-axis) and the increase in temperature in centigrade (at the X-axis)[46]. Figure 3 represents the thermal degradation of all hydrogels. The initial phase involves the loss of weight due to the evaporation of moisture and bound water, occurring between 24 °C and 240 °C[61]. In hydrophilic polymers, three distinct types of absorbed water are recognized: freezing bound water, free water, and bound/non-freezing water. Free water, without any significant interactions, evaporates completely by 100 °C. Meanwhile, freezing water forms weak bonds with polymer chains, and bound/non-freezing water forms hydrogen bonds with the polymer chains. Consequently, these two types of water are liberated from the polymer structure above 100 °C[62-64]. During this phase, any remaining formic acid may also be removed. The second stage hinges on the breakdown of the primary structure of the hydrogel matrix, known as the initiation of degradation, commencing approximately at 240 °C, with degradation ceasing around 400 °C[65]. During this phase, the dissolution of the feeble connections between the polymer chains within the hydrogels occurs[66]. In the third and ultimate stage, from 350 to 600 °C, the entire hydrogel structure breaks down into smaller fragments[67]. During the first onset, HPCG2 lost 30% at 120 °C. HPCG1 showed a 26% weight reduction at 114 °C. On the other hand, the weight loss in APCG2 and APCG1 was noticed as 26% at 123 °C and 20% at 115 °C respectively. The honey-containing hydrogels represented lesser weight loss than the aloe vera-containing hydrogels. The control film showed the lowest rate of degradation among all hydrogels. All hydrogels displayed complete degradation around 520 °C and exhibited good thermal stability.
3.3 Swelling in distilled water
Depending upon the hydrogel material, they show different swelling behaviours towards moisture. The hydrogels tend to absorb solvents like water and increase the volume. The swelling response of prepared hydrogels was checked against distilled water in terms of "change in weight as a function of time." Figure 4 represents an increasing trend in the swelling of the hydrogels with time. HPCG2 (50 min equilibrium time) showed more swelling than HPCG1 (60 min equilibrium time). The APCG1 (60 min equilibrium time) expressed a higher swelling rate than APCG2 (60 min equilibrium time). The APCG gels exhibited a higher rate of swelling than the swelling degree of HPCG gels. The reason is that aloe vera possesses polysaccharides like acemannan (β-1,4 acetylated mannan), glucomannan, and pectic acid, which enhance its hydrophilic nature and capacity to retain water. Consequently, this results in greater swelling ratios in hydrogel compositions. Additionally, aloe vera helps to relax the polymer network, thus enabling fluid penetration between polymeric chains, thereby enhancing swelling capacity[68,69]. On the contrary, hydrogels made with honey demonstrate lower swelling ratios because they are predominantly composed of sugars, namely glucose, and fructose, which have lower hydrophilicity when compared to the polysaccharides present in aloe vera. Furthermore, the viscosity of honey also plays a significant role in influencing the swelling process[70,71]. The control film PCG (0% aloe vera and honey) showed a 50 min equilibrium time and reported maximum swelling capacity compared to HPCG and APCG hydrogels.
3.4 Swelling response to varying pH
The swelling response of all hydrogels was examined against buffer solutions of pH 2, 4, 6, 7 and 8. The pre-weighed samples of hydrogels were immersed into each buffer solution for equilibrium time, and swelling was determined using equation 2.1.
The data from the swelling analysis is presented in Figure 5. The equilibrium time of PCG and HPCG2 was 50 min, while HPCG1, APCG1, and APCG2 showed 60 min as the equilibrium time for maximum swelling. Both the variables, pH and swelling, exhibited an inverse relation. With an increase in the pH of the buffer solution, a clear decrease in the swelling of all crosslinked hydrogels was noted. The APCG hydrogels displayed a higher degree of swelling in an acidic medium (pH = 2-2.2), while the swelling rate of HPCG hydrogels was comparatively low.
At acidic pH, all the hydrogels were reported to have maximum swelling compared to neutral and basic pH. All hydrogels are composed of chitosan with hydroxyl and amino groups with specific pKa values. At a pH value lower than the pKa value, the hydroxyl and amino groups remain protonated, allowing the solvent to penetrate the polymer network, which causes increased swelling. At acidic pH, the amino groups get additional protons and become ammonium ions (-NH3+), which causes charge repulsion and a decrease in osmotic pressure, leading to the movement of solvent from the solution into the polymer matrix. However, at pH equal to or higher than the pKa value of pendent groups, more than half of the hydroxyl and amino groups get deprotonated, causing decreased swelling due to reduced polymer chain interactions[72].
4. Conclusions
Polymer-based crosslinked hydrogels were prepared by physical blending and solution casting methods using CS, PEG, GPTMS, honey, and aloe vera gel. The concentration of honey and aloe vera was varied to develop two series of films. FTIR analysis verified the presence of characteristic functional groups in all materials. The emergence of peaks at 1,576, 1,110, 1,230, 1,280, and 1,070 cm-1 showed successful incorporation of CS, PEG, and GPTMS in all samples. All hydrogels were found thermally stable. APCG2 possessed the highest stability among all. The fabricated hydrogels showed considerable swelling in distilled water and exhibited pH responsiveness. The PCG showed maximum swelling in water in 60 minutes, while APCG hydrogels displayed higher swelling in the acidic pH. Although the study is constrained by the absence of biological or medical tests, the outcomes from various characterizations and analyses suggest that chitosan/PEG/GPTMS-based hydrogels with honey or aloe vera could be suitable for wound dressing and other biomedical applications.
Acknowledgment
The authors are thankful for the contributions of the School of Chemistry, University of Punjab Lahore, and the Department of Chemistry, University of Narowal.
Author contributions
Hanif S: Writing-original draft, visualization, software.
Rehmat S: Project administration, investigation, conceptualization.
Hassan M, Khan RU: Review.
Majeed F: Data curation, visualization.
Islam A: Conceptualization, investigation, supervision.
Conflict of interest
The authors have no conflict of interest.
Ethical approval
Not applicable.
Consent to participate
Not applicable.
Consent for publication
Not applicable.
Availability of data and materials
Not applicable.
Funding
None.
Copyright
© The Author(s) 2024.
References
-
1. Rapa M, Darie-Nita RN. Polymers in wound dressing. In: Thomas S, Tharayil A, editors. Polymeric Materials for Biomedical Implants. Amsterdam: Elsevier; 2024. p. 149-89.
[DOI] -
2. Naghib SM, Amiri S, Mozafari MR. Stimuli-responsive chitosan-based nanocarriers for drug delivery in wound dressing applications: a review. Carbohydr Polym Technol Appl. 2024;7(3):100497.
[DOI] -
3. Zhang X, Wang Y, Gao Z, Mao X, Cheng J, Huang L, et al. Advances in wound dressing based on electrospinning nanofibers. J Appl Polym Sci. 2024;141(1):e54746.
[DOI] -
4. Gultekin HE, Yasayan G, Bal-Oztürk A, Bigham A, Simchi A, Zarepour A, et al. Advancements and applications of upconversion nanoparticles in wound dressings. Mater Horiz. 2024;11(10):363-87.
[DOI] -
5. Pourjavadi A, Heydarpour R, Tehrani ZM. Multi-stimuli-responsive hydrogels and their medical applications. New J Chem. 2021;45(35):15705-15717.
[DOI] -
6. Fuchs S, Shariati K, Ma M. Specialty tough hydrogels and their biomedical applications. Adv Healthc Mater. 2020;9(2):e1901396.
[PubMed] [PMC] -
7. Liang Y, He J, Guo B. Functional hydrogels as wound dressing to enhance wound healing. ACS Nano. 2021;15(8):12687-12722.
[DOI] -
8. Manzoor A, Dar AH, Pandey VK, Shams R, Khan S, Panesar PS, et al. Recent insights into polysaccharide-based hydrogels and their potential applications in food sector: a review. Int J Biol Macromol. 2022;213(2):987-1006.
[DOI] -
9. Wei W, Ma Y, Yao X, Zhou W, Wang X, Li C, et al. Advanced hydrogels for the repair of cartilage defects and regeneration. Bioact Mater. 2020;6(4):998-1011.
[DOI] -
10. Majeed F, Razzaq A, Rehmat S, Azhar I, Mohyuddin A, Rizvi NB. Enhanced dye sequestration with natural polysaccharides-based hydrogels: a review. Carbohydr Polym. 2024;330:121820.
[DOI] -
11. Tchemtchoua VT, Atanasova G, Aqil A, Filee P, Garbacki N, Vanhooteghem O, et al. Development of a chitosan nanofibrillar scaffold for skin repair and regeneration. Biomacromolecules. 2011;12(9):3194-3204.
[DOI] -
12. Oryan A, Sahvieh S. Effectiveness of chitosan scaffold in skin, bone and cartilage healing. Int J Biol Macromol. 2017;104(Pt A):1003-1011.
[DOI] -
13. Khan A, Alamry KA. Recent advances of emerging green chitosan-based biomaterials with potential biomedical applications: a review. Carbohydr Res. 2021;506:108368.
[DOI] -
14. Niculescu AG, Grumezescu AM. Applications of chitosan-alginate-based nanoparticles-an up-to-date review. Nanomaterials. 2022;12(2):186.
[DOI] -
15. Khan MA, Mujahid M. A review on recent advances in chitosan based composite for hemostatic dressings. Int J Biol Macromol. 2019;124(1):138-147.
[DOI] -
16. Kean T, Thanou M. Biodegradation, biodistribution and toxicity of chitosan. Adv Drug Deliv Rev. 2010;62(1):3-11.
[DOI] -
17. Bano I, Arshad M, Yasin T, Ghauri MA, Younus M. Chitosan: a potential biopolymer for wound management. Int J Biol Macromol. 2017;102:380-383.
[DOI] -
18. Gao Y, Wu Y. Recent advances of chitosan-based nanoparticles for biomedical and biotechnological applications. Int J Biol Macromol. 2022;203(1-2):379-388.
[DOI] -
19. Lv S, Zhang S, Zuo J, Liang S, Yang J, Wang J, et al. Progress in preparation and properties of chitosan-based hydrogels. Int J Biol Macromol. 2023;242(Pt 2):124915.
[DOI] -
20. Hamedi H, Moradi S, Hudson SM, Tonelli AE, King MW. Chitosan based bioadhesives for biomedical applications: a review. Carbohydr Polym. 2022;282:119100.
[DOI] -
21. Zhao J, Qiu P, Wang Y, Wang Y, Zhou J, Zhang B, et al. Chitosan-based hydrogel wound dressing: from mechanism to applications, a review. Int J Biol Macromol. 2023;244:125250.
-
22. Ou Y, Tian M. Advances in multifunctional chitosan-based self-healing hydrogels for biomedical applications. J Mater Chem B. 2021;9(38):7955-7971.
-
23. Sun G, Zhang XZ, Chu CC. Effect of the molecular weight of polyethylene glycol (PEG) on the properties of chitosan-PEG-poly(N-isopropylacrylamide) hydrogels. J Mater Sci Mater Med. 2008;19(8):2865-2872.
[DOI] -
24. Tirino P, Laurino R, Maglio G, Malinconico M, d'Ayala GG, Laurienzo P. Synthesis of chitosan-PEO hydrogels via mesylation and regioselective Cu(I)-catalyzed cycloaddition. Carbohydr Polym. 2014;112:736-745.
[DOI] -
25. Shi J, Wang D, Wang H, Yang X, Gu S, Wang Y, et al. An injectable hemostatic PEG-based hydrogel with on-demand dissolution features for emergency care. Acta Biomater. 2022;145:106-121.
[DOI] -
26. Li W, Zhan P, De Clercq E, Lou H, Liu X. Current drug research on PEGylation with small molecular agents. Prog Polym Sci. 2013;38(3-4):421-444.
[DOI] -
27. Xiao Z, Zhao S, Zhang X, Gang W, Su Z. Recent advances in peptide engineering of PEG hydrogels: strategies, functional regulation, and biomedical applications. Macromol Mater Eng. 2022;307(11):2200385.
[DOI] -
28. Wang Z, Ye Q, Yu S, Akhavan B. Poly ethylene glycol (PEG)-based hydrogels for drug delivery in cancer therapy: a comprehensive review. Adv healthcare Mater. 2023;12(18):2300105.
[DOI] -
29. Dethe MR, Prabakaran A, Ahmed H, Agrawal M, Roy U, Alexander A. PCL-PEG copolymer based injectable thermosensitive hydrogels. J Controlled Release. 2022;343(Pt 1):217-236.
[DOI] -
30. Brown A, He H, Trumper E, Valdez J, Hammond P, Griffith LG. Engineering PEG-based hydrogels to foster efficient endothelial network formation in free-swelling and confined microenvironments. Biomaterials. 2020;243:119921.
[DOI] -
31. Shi J, Yu L, Ding J. PEG-based thermosensitive and biodegradable hydrogels. Acta Biomater. 2021;128:42-59.
[DOI] -
32. Stewart A, Schlosser B, Douglas EP. Surface modification of cured cement pastes by silane coupling agents. ACS Appl Mater Interfaces. 2013;5(4):1218-1225.
[DOI] -
33. Li CP, Weng MC, Huang SL. Preparation and characterization of pH sensitive chitosan/3-glycidyloxypropyl trimethoxysilane (GPTMS) hydrogels by Sol-Gel method. Polymers. 2020;12(6):1326.
[DOI] -
34. Connell LS, Gabrielli L, Mahony O, Russo L, Cipolla L, Jones JR. Functionalizing natural polymers with alkoxysilane coupling agents: reacting 3-glycidoxypropyl trimethoxysilane with poly (γ-glutamic acid) and gelatin. Polym Chem. 2017;8(6):1095-1103.
[DOI] -
35. Sohrabi M, Yekta BE, Rezaie HR, Naimi-Jamal MR. The influence of 3-glycidyloxypropyl trimethoxysilane on the rheological and in-vitro behavior of injectable composites containing 64S bioactive glass, chitosan, and gelatin. J Appl Polym Sci. 2021;138(38):50963.
[DOI] -
36. Subramanian R, Hebbar HU, Rastogi NK. Processing of honey: a review. Int J Food Prop. 2007;10(1):127-143.
[DOI] -
37. Kumar KPS, Bhowmik D, Chiranjib, Biswajit, Chandira MR. Medicinal uses and health benefits of honey: an overview. J Chem Pharm Res. 2010;2(1):385-395. Available from: https://www.renevanmaarsseveen.nl/wp-content/uploads/overig4.pdf
-
38. Iqbal DN, Munir A, Abbas M, Nazir A, Ali Z, Alshawwa SZ, et al. Polymeric membranes of Chitosan/Aloe vera gel fabrication with enhanced swelling and antimicrobial properties for biomedical applications. Dose Response. 2023;21(2):15593258231169387.
[DOI] -
39. Maan AA, Nazir A, Khan MKI, Ahmad T, Zia R, Murid M, et al. The therapeutic properties and applications of Aloe vera: a review. J Herb Med. 2018;12:1-10.
[DOI] -
40. Sanchez M, Gonzalez-Burgos E, Iglesias I, Gomez-Serranillos MP. Pharmacological update properties of Aloe vera and its major active constituents. Molecules. 2020;25(6):1324.
[DOI] -
41. Sharma P, Kharkwal AC, Kharkwal H, Abdin MZ, Varma A. A review on pharmacological properties of Aloe vera. Int J Pharm Sci Rev Res. 2014;29(2):31-37. Available from: https://globalresearchonline.net/07.pdf
-
42. Nguyen HTL, Panyoyai N, Paramita VD, Mantri N, Kasapis S. Physicochemical and viscoelastic properties of honey from medicinal plants. Food Chem. 2018;241:143-149.
[DOI] -
43. Li C, Jiang T, Zhou C, Jiang A, Lu C, Yang G, et al. Injectable self-healing chitosan-based POSS-PEG hybrid hydrogel as wound dressing to promote diabetic wound healing. Carbohydr Polym. 2023;299:120198.
[DOI] -
44. Chen RN, Wang GM, Chen CH, Ho HO, Sheu MT. Development of N,O-(carboxymethyl)chitosan/collagen matrixes as a wound dressing. Biomacromolecules. 2006;7(4):1058-1064.
[DOI] -
45. Hasan MM, Uddin MF, Zabin N, Shakil MS, Alam M, Achal FJ, et al. Fabrication and characterization of chitosan-polyethylene glycol (Ch-Peg) based hydrogels and evaluation of their potency in rat skin wound model. Int J Biomater. 2021;2021(1):4877344.
[DOI] -
46. Rasool A, Ata S, Islam A. Stimuli responsive biopolymer (chitosan) based blend hydrogels for wound healing application. Carbohydr. Polym. 2019;203:423-429. doi:
[DOI] -
47. Gull N, Khan SM, Butt OM, Islam A, Shah A, Jabeen S, et al. Inflammation targeted chitosan-based hydrogel for controlled release of diclofenac sodium. Int J Biol Macromol. 2020;162:175-187.
[DOI] -
48. Rehmat S, Rizvi NB, Khan SU, Ghaffar A, Islam A, Khan RU, et al. Novel stimuli-responsive pectin-PVP-functionalized clay based smart hydrogels for drug delivery and controlled release application. Front Mater. 2022;9:823545.
[DOI] -
49. Sudarsan S, Franklin DS, Sacthivel M, Guhanathan S. Non toxic, antibacterial, biodegradable hydrogels with pH-stimuli sensitivity: Investigation of swelling parameters. Carbohydr Polym. 2016;148:206-215.
[DOI] -
50. Sharma R, Kaith BS, Kalia S, Pathania D, Kumar A, Sharma N, et al. Biodegradable and conducting hydrogels based on Guar gum polysaccharide for antibacterial and dye removal applications. J Environ Manage. 2015;162:37-45.
[DOI] -
51. Butt A, Jabeen S, Nisar N, Islam A, Gull N, Iqbal SS, et al. Controlled release of cephradine by biopolymers based target specific crosslinked hydrogels. Int J Biol Macromol. 2019;121(3):104-112.
[DOI] -
52. Andleeb A, Mehmood A, Tariq M, Butt H, Ahmed R, Andleeb A, et al. Hydrogel patch with pretreated stem cells accelerates wound closure in diabetic rats. Biomater Adv. 2022;142(6):213150.
[DOI] -
53. Ghauri ZH, Islam A, Qadir MA, Ghaffar A, Gull N, Azam M, et al. Novel pH-responsive chitosan/sodium alginate/PEG based hydrogels for release of sodium ceftriaxone. Mater Chem Phys. 2022;277:125456.
[DOI] -
54. Sudarsan S, Selvi MS, Chitra G, Sakthivel S, Franklin DS, Guhanathan S. Nontoxic pH-sensitive silver nanocomposite hydrogels for potential wound healing applications. Polym-Plast Technol Mater. 2021;60(1):84-104.
[DOI] -
55. Jabeen S, Islam A, Ghaffar A, Gull N, Hameed A, Bashir A, et al. Development of a novel pH sensitive silane crosslinked injectable hydrogel for controlled release of neomycin sulfate. Int J Biol Macromol. 2017;97:218-227.
[DOI] -
56. Li X, Wang Y, Li A, Ye Y, Peng S, Deng M, et al. A novel pH- and salt-responsive N-succinyl-chitosan hydrogel via a one-step hydrothermal process. Molecules. 2019;24(23):4211.
[DOI] -
57. Tork BM, Nejad NH, Ghalehbagh S, Bashari A, Shakeri-Zadeh A, Kamrava SK. In situ green synthesis of silver nanoparticles/chitosan/poly vinyl alcohol/poly ethylene glycol hydrogel nanocomposite for novel finishing of nasal tampons. J Ind Text. 2016;45(6):1399-1416.
[DOI] -
58. Saberian M, Seyedjafari E, Zargar SJ, Mahdavi FS, Sanaei-rad P. Fabrication and characterization of alginate/chitosan hydrogel combined with honey and aloe vera for wound dressing applications. J Appl Polym Sci. 2021;138(47):51398.
[DOI] -
59. Chopra H, Bibi S, Kumar S, Khan MS, Kumar P, Singh I. Preparation and evaluation of chitosan/PVA based hydrogel films loaded with honey for wound healing application. Gels. 2022;8(2):111.
[DOI] -
60. Mukhopadhyay A, Rajput M, Barui A, Chatterjee SS, Pal NK, Chatterjee J, et al. Dual cross-linked honey coupled 3D antimicrobial alginate hydrogels for cutaneous wound healing. Mater Sci Eng C Mater Biol Appl. 2020;116:111218.
[DOI] -
61. Sudarsan S, Trofimov E, Franklin DS, Venthan SM, Guhanathan S, Mavinkere Rangappa, et al. Thermal, morphology and bacterial analysis of pH-responsive sodium carboxyl methylcellulose/ fumaric acid/ acrylamide nanocomposite hydrogels: synthesis and characterization. Heliyon. 2023;9(11):e20939.
[DOI] -
62. Altinisik A, Yurdakoc K. Synthesis, characterization, and enzymatic degradation of chitosan/PEG hydrogel films. J Appl Polym Sci. 2011;122(3):1556-1563.
[DOI] -
63. Gierszewska-Druzynska M, Ostrowska-Czubenko J. The effect of ionic crosslinking on thermal properties of hydrogel chitosan membranes. Prog Chem Appl Chitin Its Deriv. 2010;15:25-32. Available from: https://bibliotekanauki.pl/articles/1035522.pdf.
-
64. Rao Z, Liu S, Wu R, Wang G, Sun Z, Bai L, et al. Fabrication of dual network self-healing alginate/guar gum hydrogels based on polydopamine-type microcapsules from mesoporous silica nanoparticles. Int J Biol Macromol. 2019;129:916-926.
[DOI] -
65. Sarwar MS, Ghaffar A, Islam A, Yasmin F, Oluz Z, Tuncel E, et al. Controlled drug release behavior of metformin hydrogen chloride from biodegradable films based on chitosan/poly (ethylene glycol) methyl ether blend. Arabian J Chem. 2020;13(1):393-403.
[DOI] -
66. Martinez-Martinez M, Rodriguez-Berna G, Bermejo M, Gonzalez-Alvarez I, Gonzalez-Alvarez M, Merino V. Covalently crosslinked organophosphorous derivatives-chitosan hydrogel as a drug delivery system for oral administration of camptothecin. Eur J Pharm Biopharm. 2019;136:174-183.
[DOI] -
67. Tanan W, Panichpakdee J, Saengsuwan S. Novel biodegradable hydrogel based on natural polymers: Synthesis, characterization, swelling/reswelling and biodegradability. Eur Polym J. 2019;112(181):678-687.
[DOI] -
68. Bialik-Was K, Pluta K, Malina D, Barczewski M, Malarz K, Mrozek-Wilczkiewicz A. Advanced SA/PVA-based hydrogel matrices with prolonged release of Aloe vera as promising wound dressings. Mater Sci Eng C Mater Biol Appl. 2021;120:111667.
[DOI] -
69. Irfan J, Ali A, Hussain MA, Haseeb MT, Naeem-ul-Hassan M, Hussain SZ. Citric acid cross-linking of a hydrogel from Aloe vera (Aloe barbadensis M.) engenders a pH-responsive, superporous, and smart material for drug delivery. RSC Adv. 2024;14(12):8018-8027.
[DOI] -
70. El-Kased RF, Amer RI, Attia D, Elmazar MM. Honey-based hydrogel: In vitro and comparative In vivo evaluation for burn wound healing. Sci Rep. 2017;7(1):9692.
[DOI] -
71. Azam NANM, Amin KAM. Influence of Manuka Honey on Mechanical Performance and Swelling Behaviour of Alginate Hydrogel Film. IOP Conf Ser: Mater Sci Eng. 2018;440(1):012024.
[DOI] -
72. Ghauri ZH, Islam A, Qadir MA, Gull N, Haider B, Khan RU, et al. Development and evaluation of pH-sensitive biodegradable ternary blended hydrogel films (Chitosan/Guar gum/PVP) for drug delivery application. Sci Rep. 2021;11(1):21255.
[DOI]
Copyright
© The Author(s) 2024. This is an Open Access article licensed under a Creative Commons Attribution 4.0 International License (https://creativecommons.org/licenses/by/4.0/), which permits unrestricted use, sharing, adaptation, distribution and reproduction in any medium or format, for any purpose, even commercially, as long as you give appropriate credit to the original author(s) and the source, provide a link to the Creative Commons license, and indicate if changes were made.
Publisher’s Note
Share And Cite