Abstract
Peptic ulcer is a common disease with a high clinical incidence and frequent recurrences. The well-characterized galactomannan-type polysaccharide, extracted from Cassia grandis seeds, can accelerate the stages of wound healing and improve the remodeling of the extracellular matrix of injured tissues; this way, the gastroprotective potential of the galactomannan was proposed to be evaluated in the treatment of rat peptic ulcers induced by ethanol. Galactomannan was extracted by ethanol precipitation and prepared at 0.1, 1.0, and 10 mg/kg concentrations. Male Wistar rats were randomly divided into groups (n = 5) and orally treated with saline (negative control, NC), galactomannan at 0.1 mg/Kg (G0.1), galactomannan at 1.0 mg/Kg (G1), galactomannan at 10 mg/Kg (G10), and lansoprazole 30 mg/Kg (positive control, CP). Ethanol (1 mL/100 g of body mass) was administered 1 h after the treatments and, after another hour, the animals were euthanized. The stomachs were removed, fixed between Petri dishes, and photographed to calculate the percentage of the injured regions by computerized software. Histological analysis and the quantification of lipid peroxidation and reduced glutathione (GSH) were also performed. G1 and G10 reduced the injured area (P < 0.001) by 40 and 36%, respectively, concerning NC. It was observed the preservation of the gastric mucosa in all of the animals treated with galactomannan, in addition to reduced (P < 0.001) lipid peroxidation and GSH levels higher (P < 0.05) than NC. The galactomannan extracted from C. grandis seeds showed gastroprotective activity at 1.0 and 10 mg/kg by reducing the area of ulceration and oxidative stress.
Keywords
1. Introduction
Polysaccharides are natural polymers biosynthesized by plants, animals, and microorganisms and characterized by the union of many monosaccharide units, of the same type or not, linked by glycosidic bonds in both linear and branched conformations[1]. Being classified as gums or mucilages, polysaccharides have been well recognized by properties of biocompatibility, low cost of extraction, bioavailability, and non or low toxicity[2]. Starch, cellulose, chitin, agar, alginate, pectin, galactomannan, and xyloglucan are the main polysaccharides reported in the scientific literature, presenting excellent results in the food[3,4], biomedical[5,6] and cosmetics[7,8]. Regarding its biological activities, recent studies have demonstrated the healing[9], antioxidant[10,11], antitumor[12], anticoagulant[13], antiviral[14], and immunomodulatory[15,16] potential of polysaccharides from different sources.
Galactomannans are polysaccharides formed by a linear chain composed of β-1,4-D-mannopyranose units replaced by α-1,6-D-galactopyranose units. They are widely found in the endosperm of plant seeds, especially in members of the Leguminosae family, where they perform an energy reserve function[17]. This family has species spread throughout the world, especially in tropical and subtropical regions; in Brazil, the galactomannan extracted from Cassia grandis seeds collected in the northeast region was extensively characterized[18] and formulated as a matrix for the immobilization of biomolecules[19,20], film for incorporating nanoparticles[21], substitute for fat in cakes[22], and healing agent[9,20].
Peptic ulcer is a common disease, with a high clinical incidence and generally frequent recurrences; it is estimated that 10% of adults are affected by the disease at least once throughout their lives[23]. According to Kavitt et al.[24], stress is the etiology of peptic ulcers since gastric acid production is dangerously increased in this condition. In this context, addictions such as smoking and alcohol consumption, prolonged use of non-steroidal anti-inflammatory drugs, and bacterial infection caused by Helicobacter pylori can be decisive in the disease emergence or complication[25].
The reference treatment for peptic ulcers is carried out with proton pump inhibitors[26], suppressing hydrochloric acid while the underlying cause of the disease is resolved. Despite the relevant cure rate for this disease, the treatment is expensive and commonly related to side effects ranging from uncomfortable to serious, such as hypergastrinemia, hypochlorhydria, and problems in the absorption of essential nutrients[27]. These factors may culminate in low adherence to therapy as well as the difficulty of treatment for low-income populations[24].
Research into new and natural forms of treatment contributes to the strengthening of scientific knowledge through the accumulation of know-how and the consolidation of a sustainable economy. These characteristics are extremely relevant as they suggest new alternatives to natural treatments and the softening of environmental problems. Due to its versatility and especially its ability to accelerate the stages of wound healing and improve the remodeling of the extracellular matrix of injured tissues, the galactomannan obtained from C. grandis seeds has the potential to be explored in the treatment of peptic ulcers in experimental models, therefore being the primary objective of this work. A summarized scheme with the main contents of this research, including the basis behind the use of the galactomannan from C. grandis, its intrinsic characteristics, the suggestion for its application as gastroprotective agent in experimental models, the main findings of this work, and future perspectives of our research group can be seen in Figure 1.
2. Materials and Methods
2.1 Extraction of the galactomannan from C. grandis seeds and preparation of polysaccharide solutions
grandis seeds were collected in the agreste region of Pernambuco (Brazil) and processed according to the methodology of Albuquerque et al.[18]. 50.34 g of seeds were boiled for 1 h and crushed in 1,000.8 mL of 0.1 M NaCl. The solution was filtered through a screen-printing cloth and the filtrate was subjected to precipitation with 46% (v/v) ethanol 1:3 (v/v) for 18 h. The precipitate was separated from the supernatant through filtration on a screen-printing cloth and subjected to two washes with absolute ethanol for 30 min and one wash with acetone for the same period. Between each wash, the precipitate was filtered with a screen-printing cloth, and the supernatant was discarded. Finally, the precipitate was dried in an oven at 40 ºC, crushed using a pestle, and called galactomannan.
According to Albuquerque et al.[18], rheological studies revealed that the 1.6% (w/v) concentration represents the solution-gel transition for this galactomannan; considering this information and other studies using polysaccharides as gastroprotective agents against induced peptic ulcers in experimental models[28,29], aqueous solutions of the galactomannan were prepared at 0.1, 1.0, and 10 mg/kg concentrations under constant magnetic stirring (250 rpm), for 12-24 h, at room temperature (22 ± 2 ºC). The solutions were refrigerated (2 to 8 ºC) until in vivo experiments.
2.2 Animals
In vivo experiments were performed with male Wistar rats (Rattus norvegicus) aged 2 - 3 months, weighing an average of 260 g, from the vivarium of the Department of Antibiotics at the Federal University of Pernambuco (UFPE). The animals were maintained under controlled lighting conditions (12 h light/dark cycle) and temperature (22 ± 2 ºC), and received water and food (Presence®) ad libitum. All experimental protocols were approved by the Ethics Committee on the Use of Animals of the Federal Rural University of Pernambuco (process number 2823030920).
2.3 Induction of gastric ulcer by absolute ethanol
The induction of gastric ulcer and the dose used in group 5 (positive control, PC) were performed as described by Almeida el al.[30]. After fasting for 14 h, the animals were divided into groups (n = 5), treated orally, and classified as follows: group 1 (negative control-NC), treated with distilled water (3 mL/100 g of body mass); groups 2 (G0.1), 3 (G1), and 4 (G10), treated with aqueous solutions of the galactomannan at 0.1, 1.0, and 10.0 mg/kg concentrations, respectively; and group 5 (positive control, PC), treated with 30 mg/kg of lansoprazole. Ethanol (1 mL/100 g of body mass) was administered orally 1 h after the treatments for all of the groups, and the animals were euthanized with a lethal dose of sodium thiopental (200 mg·kg -1) 1 h after the ethanol administration. The stomachs were removed, washed with distilled water, and fixed between Petri dishes to be photographed. The hemorrhagic areas of the gastric mucosa were measured and the percentage of damaged area was calculated using computerized software (ImageJ, National Institutes of Health, 9000 Rockville Pike, Bethesda, Maryland, USA). The results (%) were expressed as the total area of ulcerative lesion in mm2 (A lesion) to the total area of the gastric body (A stomach), as expressed in Eq. (1).
2.4 Histological analysis
The stomachs were fixed in 10 % (v/v) formalin for 24 h, dehydrated with ethyl ethanol, cleared with xylene, embedded in a Histosec® kit, and prepared for microtomy. 5 mm samples were deparaffinized, rehydrated with ethyl ethanol, and subsequently stained with hematoxylin-eosin. Lesions were analyzed using a Micro Dip® automatic microscopy system (Kacil Inc.).
2.5 Evaluation of the oxidative stress
The gastric mucosa of the animals was homogenized in a buffer solution of 1.15 % KCl with 3 mM EDTA (5 mL/g of tissue) to evaluate the oxidative stress using 2 different methods. The quantification of lipid peroxidation was performed using the method of Ohkawa et al.[31], and levels of reduced glutathione (GSH) were measured using the method of Sedlak and Lindsay[32]. Protein measurement was performed using the BCA method[33] with 0.1% bovine serum albumin as standard.
2.6 Statistical analysis
The results obtained in this study showed a normal distribution. The Kolmogorov-Smirnov test (With Dallal-Wilkinson-Lillie for P value) was performed before the statistical analysis using analysis of variance and Student's t test (SigmaStat, 2003, USA). Values of P < 0.05 were considered significant, and statistical differences were represented by *, **, and *** respectively for P < 0.05, P < 0.01, and P < 0.001.
3. Results and Discussion
The final weight of the galactomannan powder was 17.98 g, which is equivalent to a yield of 35.74% and agrees with the yield of 36 ± 8% already reported for the same galactomannan from C. grandis seeds collected in the agreste region of Pernambuco (Brazil)[18]. Additionally, our result is similar to the one (33%) obtained for the seeds of C. grandis collected in India[34] and higher to the value of 26% reported for the seeds of Cassia javanica[35].
3.1 Macroscopic analysis of stomachs and percentage of injured area
Ethanol is well known as a necrotizing agent that quickly penetrates the gastric mucosa, causing erosion and ulcer formation due to the depletion of natural protective agents and an increase in free radicals[36]. Figure 2A expresses the results of the injured area (mm 2) concerning the treatment received by each group for ethanol-induced peptic ulcers. The animals belonging to group 1 (NC), that is, those treated with water, presented a value of 345.50 ± 25.60 mm2 of the ulcerated area; the animals treated with galactomannan at 0.1 (group 2 - G0.1), 1.0 (group 3 - G1), and 10.0 (group 4 - G10) mg/kg concentrations showed results of 326.50 ± 96.06, 202.30 ± 70.17, and 219 .30 ± 90.77 mm2, respectively. Group 5 (PC), treated with lansoprazole (30mg/kg), presented a result of 153.40 ± 29.18 mm2. The statistical analysis revealed that there was no statistical difference between groups 1 (NC) and 2 (G0.1); however, groups 3 (G1) and 4 (G10) were able to significantly reduce gastric lesions (P < 0.05) by 41% and 36%, respectively, when compared to group 1 (NC). Furthermore, group 5 (PC) was able to reduce the injured area (P < 0.001) by approximately 55.60% when compared to NC. Figure 2B demonstrates the macroscopic gastric damage induced by ethanol in groups 1 (NC), 2 (G0.1), 3 (G1), 4 (G10), and 5 (PC). From the images, it is possible to confirm the results shown in Figure 2A and, therefore, the gastroprotective effect of the galactomannan at 1.0 and 10 mg/Kg.
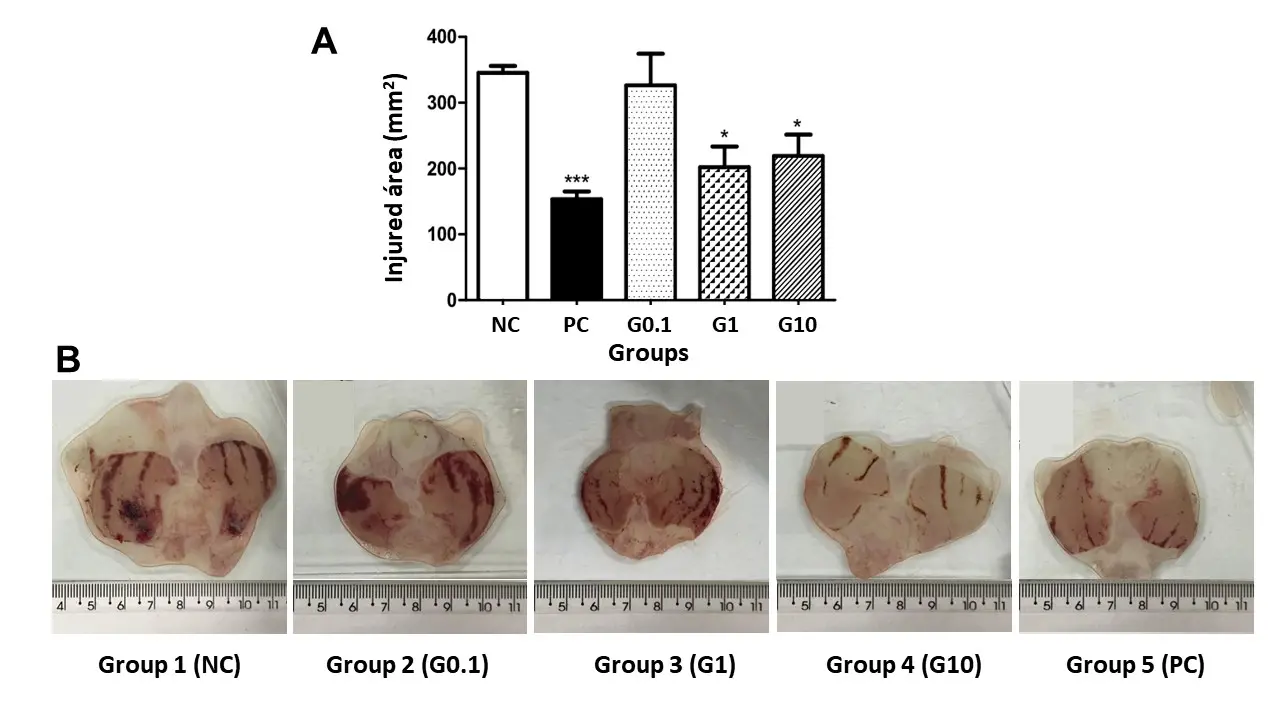
Figure 2. (A) Effect of the treatment with water, lansoprazole, and the galactomannan extracted from C. grandis seeds on ethanol-induced peptic ulcers in male rats. *P < 0.05 and ***P < 0.001 when compared to the group 1 (NC); (B) representative images of the injured area in the stomachs of rats for groups 1 (NC), 2 (G0.1), 3 (G1), 4 (G10), and 5 (PC). NC: negative control.
The literature reports some studies that analyzed the gastroprotection of natural compounds against ethanolic induction of peptic ulcers; for example, Almeida et al.[30] evaluated the anti-ulcer activity of the hexane extract of Spondias purpurea in 3 different doses and obtained higher inhibition values for the injured area than those reported in the present study. The extract at 12.5 mg/kg, close to the dose of 10 mg/kg used in this work, was able to reduce the ulcerated area by 77% when compared to the negative control. It is worth noting that, in folk medicine, several parts of S. purpurea, popularly called serigueleira, are conventionally used to treat gastric disorders due to the excellent antioxidant potential characteristic of their extracts[37]. Marques et al.[28] evaluated the anti-inflammatory and anti-ulcer activities of the galactomannan extracted from Caesalpinia pulcherrima using indomethacin as an ulcer inducer in mice. The galactomannan at 3, 10, and 30 mg/kg was able to inhibit the injured area by 48.16%, 45.71%, and 44.62%, respectively, when compared to the negative control; however, these results did not show statistical differences between them. Luo et al.[29] evaluated the gastroprotective effect of Evodiae fructus polysaccharide (EFP) against ethanol-induced gastric ulcers in mice. Similar to our results, there was no dose-dependent behavior for EFP in ameliorating ethanol-induced gastric ulcer. Compared with the negative control group, the inhibition rates on the ulcer index varied from 32 to 66%.
Another study evaluated the gastroprotective potential of the polysaccharide extracted from the seeds of Chenopodium quinoa ; in this case, the polysaccharide was able to reduce the ethanol-induced gastric lesions by 45 ± 9% and 72 ± 7% at concentrations of 30 and 100 mg/kg, respectively, compared to the negative control group[38]. These results suggested that the gastric mucosa was protected by the polysaccharide's ability to increase mucus synthesis, bind to the surface mucosa, and exert a protective coating.
The gastroprotective potential of polysaccharides extracted from different sources and other vegetable portions was already reported. For example, the arabinogalactan isolated from Cereus peruvinus gum significantly inhibited ethanol-induced gastric injuries in rats, showing an ED50 of 49 mg/kg[39]. Similar results were observed for the administration of 100 mg/kg of the galactomannoglucan extracted from the fruits of Syagrus oleracea ; in this case, a significant reduction in gastric hyperemia was observed, as well as in the severity of animals' lesions treated with the polysaccharide[40]. Finally, Cipriani et al.[41] evaluated the gastroprotective potential of acidic heteroxylans extracted from the leaves of Maytenus ilicifolia and Phyllanthus niruri in an ethanol induction model, obtaining a reduction of 65% and 78% of gastric lesions and ED50 of 40.0 and 20.4 mg/kg, respectively.
Despite the excellent biological activities demonstrated by the galactomannan from C. grandis, in what concerns the best of our knowledge, its antioxidant potential has not been reported in vitro or in vivo, which allows us to hypothesize that the gastroprotective action reported in this study is related to the ability to modulate the immune system against induced injury and accelerate the inflammatory stage of healing.
3.2 Histological analysis
Figure 3 shows the morphological and histological profile of the gastric mucosa of the rats treated with water, lansoprazole, and the galactomannan extracted from C. grandis seeds. It is important to mention that the ethanol used for the induction of gastric ulcer penetrates the gastric mucosa, exposing the mucous layer to hydrochloric acid and pepsin, which have proteolytic and hydrolytic effects that damage cell membranes, causing cell exfoliation, tissue erosion, and ulcer[42]. Group 1 (NC), treated with water, showed consistent damage to the gastric mucosa, with loss of the stomach's glandular structure, disorganized glandular structure, congestion, and submucosal edema (Figure 3A,B,C). Group 5 (PC), treated with lansoprazole, showed a reduction in the area of damaged epithelium and congestion; however, it was still possible to observe an extensive loss of the stomach's glandular structure, large amounts of disorganized glandular structure, and submucosal edema (Figure 3D,E,F). The above-mentioned characteristics were significantly alleviated in the groups treated with galactomannan (Figure 3G,H,I); the glandular structure was significantly less disorganized when compared to group 1 (NC) and even than in group 5 (PC). There was also a reduction in venous congestion and submucosal and cellular edema at all dosages of galactomannan. Extensive areas of preserved epithelium were visualized through histology, which is consistent with the results of reduced lipid peroxidation demonstrated in the following topic. Compared to the study developed by Marques et al.[28], in which the antiulcerogenic activity of the galactomannan from C. pulcherrima was analyzed with indomethacin as an ulcer inducer, it was observed that the preservation of the gastric mucosa was similar at 10mg/kg, in which a greater amount of normal mucosa was found when compared to the group that received only indomethacin. Other polysaccharides already studied by its gastroprotective effect also showed histological analysis; for example, the ones extracted from the seeds of C. quinoa[38] and Trigonella foenum graecum reported a considerable decrease in hyperemia, submucous edema, and venous congestion in acute injuries induced by ethanol in experimental models[43].
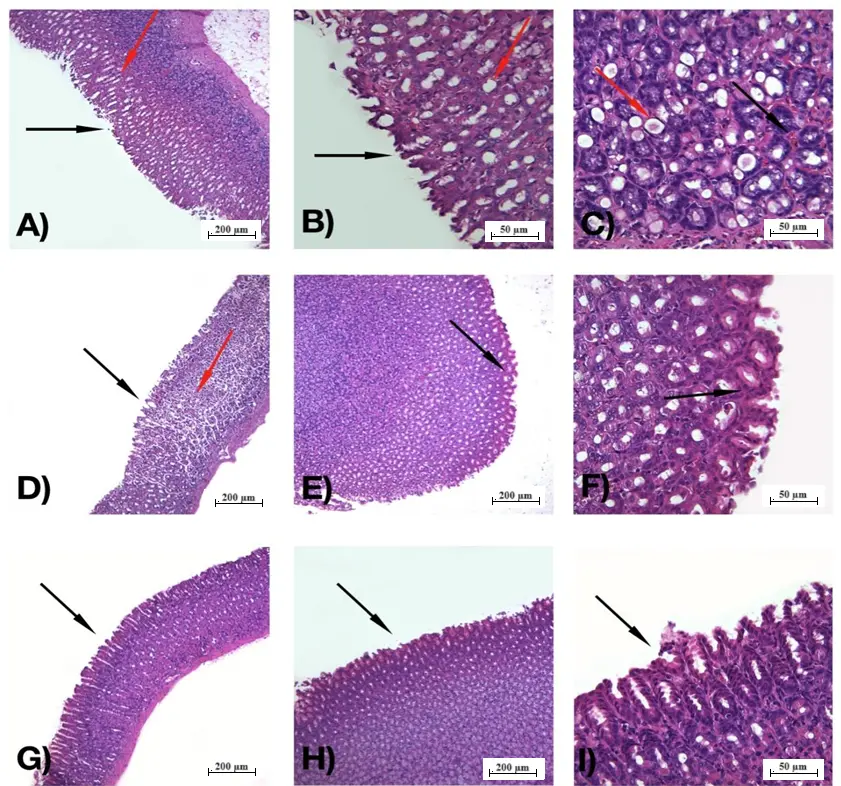
Figure 3. Histological morphology of the gastric mucosa of rats. (A) 100x magnification, group 1 (NC), presence of degraded epithelium and disorganized glandular structure (black arrow) and submucosal edema (red arrow); (B) 400x magnification, group 1 (NC), degraded epithelium and disorganized glandular structure (black arrow), and submucosal edema (red arrow); (C) 400x magnification, group 1 (NC), congestion (red arrow) and submucosal edema (black arrow); (D) 100x magnification, group 5 (PC), presence of degraded epithelium, with disorganized glandular structure (black arrow) and cellular edema (red arrow); (E) 100x magnification, group 5 (PC), presence of preserved epithelium (black arrow); (F) 400x magnification, group 5 (PC), presence of preserved epithelium and organized glandular structure (black arrow); (G) 100x magnification, group 2 (0.1 mg/kg galactomannan), presence of large sections of preserved epithelium (black arrow) and reduction of edema and congestion; (H) 100x magnification, group 3 (1.0 mg/kg galactomannan), presence of organized glandular structure and preserved epithelium (black arrow); (I) 400x magnification, group 4 (10 mg/kg galactomannan), preserved epithelium, and organized glandular structure (black arrow). NC: negative control.
3.3 Oxidative stress
The thiobarbituric acid reactive substance assay is used to detect lipid oxidation. This assay measures malondialdehyde (MDA), a product of an unsaturated fatty acid endoperoxide resulting from the oxidation of lipid substrates. The analysis of lipid peroxidation was performed with the stomachs treated in the present study (Figure 4A); the results showed a reduction in the levels of species reactive to the thiobarbituric acid in groups 2, 3, 4, and 5, respectively G0.1 (0.120 ± 0.029), G1 (0.142 ± 0.074), G10 (0.146 ± 0.0411), and PC (0.223 ± 0.088), when compared to group 1 (NC) (0.622 ± 0.108). In this context, it is clear that the galactomannan reduced lipid peroxidation by 80.72%, 77.5%, and 76.59% at 0.1, 1.0, and 10 mg/kg, respectively. The three concentrations were efficiently protective even when compared to group 5 (PC); furthermore, this group (PC) reduced lipid peroxidation by 64.19% when compared to NC.
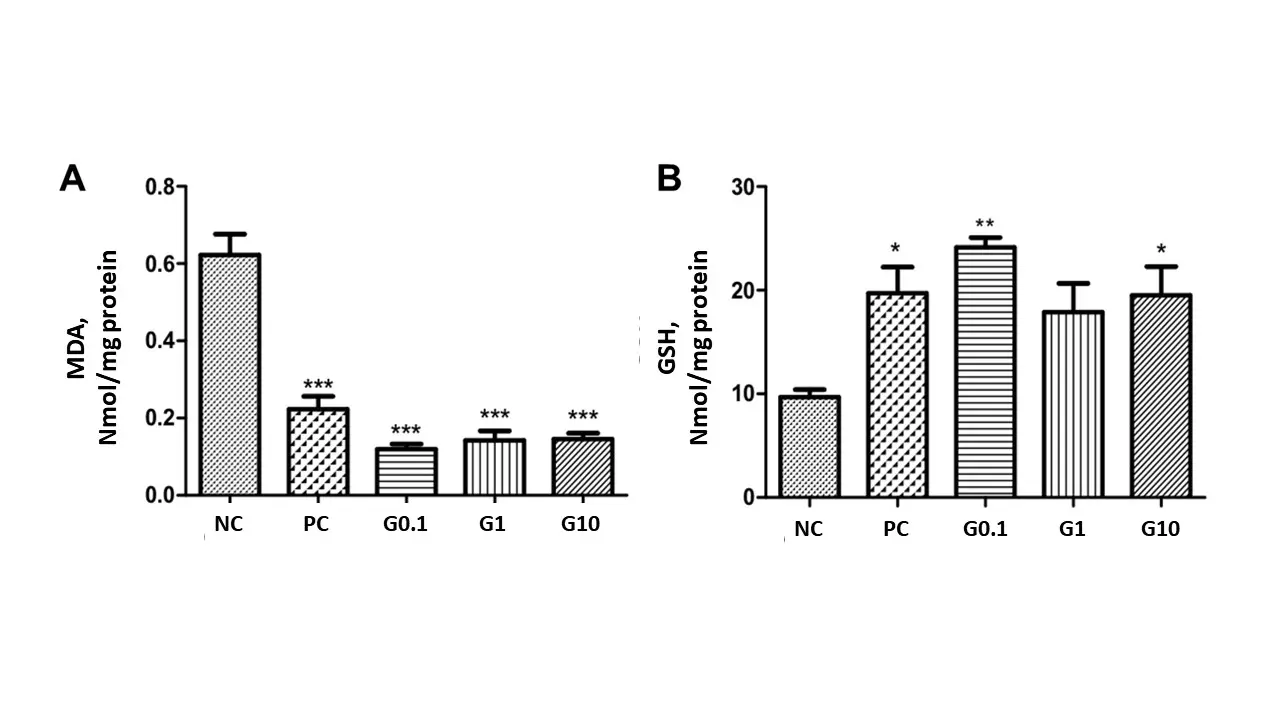
Figure 4. Effect of the treatment with the galactomannan extracted from C. grandis seeds on preventing lipid peroxidation by the TBARS method (A) and maintaining reduced glutathione concentrations (B). After fasting for 14 h, the rats were divided into groups and treated orally, according to the group to which they belong, with water, galactomannan solutions at 0.1, 1.0, and 10 mg/kg, and 30 mg/kg of lansoprazole. *P < 0.05 and ***P < 0.001 when compared to the group 1 (NC). NC: negative control.
The efficient prevention of lipid peroxidation by galactomannan can be observed in other studies reported in the scientific literature; for example, Pandian et al.[43] evaluated the gastroprotective effect of the polysaccharide extracted from fenugreek seeds (T. foenum graecum) in a model of gastric ulcer induced by ethanol. They reported that the oral administration of the polysaccharide presented significant macro and cytoprotective effects. The hypothesis is that the polysaccharide promoted an antisecretory action and prevented lipid peroxidation since the level of TBARS was significantly higher in the negative control group when compared to the treated one (P < 0.001). More recently, Sabino et al.[44] observed a 56.5% reduction in lipid peroxidation using acerola polysaccharide at 1 mg/kg, and Lian et al.[45] investigated the gastroprotective effect of Lycium barbarum polysaccharides (LBP) and C-phycocyanin (C-PC) in rats with ethanol-induced gastric ulcers. Pretreatments with LBP, C-PC, or both LBP and C-PC significantly decreased serum MDA levels compared with the ethanol-induced ulcer group (P < 0.05) by values of approximately 27%.
Glutathione, also known as GSH, is an endogenous component of cellular metabolism composed by glycine, cysteine, and glutamic acid. It is an integral part of the biotransformation of xenobiotic substances, serving as a protection to the body from oxidizing agents. Glutathione conjugation (facilitated by a glutathione transferase family of enzymes) helps the detoxification by binding electrophiles that could otherwise bind to proteins or nucleic acids, resulting in cellular damage and genetic mutations. The greater its quantity, the more protected the organism is[46]. Analyzing the preservation of GSH levels in the stomachs treated in the present work (Figure 4B), we obtained values of 9.693 ± 1.587 and 19.72 ± 5.042 μg GSH/g of tissue, respectively for groups 1 (NC) and 5 (PC), while the groups treated with galactomannan at 0.1 (G0.1), 1.0 (G1), and 10 (G10) mg/kg presented values of 24.15 ± 1.87, 17.90 ± 5.51, and 19.52 ± 6.15, respectively. The effective maintenance of GSH levels in the groups treated with galactomannan reached values of approximately 149.1% (G0.1), 84.6% (G1), and 101.3% (G10) when compared to group 1 (NC); group 5 (PC) maintained GSH levels approximately 103.4% higher than group 1 (NC). The similar results obtained for groups 4 (G10) and 5 (PC) demonstrate the effective protection of galactomannan against oxidizing agents. Yang et al.[47], for example, performed GSH analyses on the polysaccharide extracted from the Pleurotus ostreatus mushroom and obtained values almost 10 times lower than those presented in our study.
Regarding gastroprotection from a different point of view for polysaccharide applications, another study showed that boric acid regulates oxidative and inflammatory responses in the gastric mucosa and, therefore, protects the mucosa against the damage caused by ethanol[48]. The authors suggested that the gastroprotective potential of boric acid can be attributed to its regulatory function in IL-6/JAK2/STAT3 signaling pathway modulated by AMPK, in addition to the Sema3A/PlxnA1 axis and homocysteine. Another example, the administration of betaine, a glycine derivative that has three extra methyl groups, was able to significantly reduce homocysteine levels and improve the damage caused to the gastric mucosa in an experimental model of ethanol-induced peptic ulcer disease[49]. The authors observed that MDA levels decreased while GSH and catalase activity levels increased with the betaine treatment. Furthermore, they observed that betaine was able to reduce apoptosis.
Considering the above, it is possible to summarize the oxidative stress caused by the ethanolic induction of gastric ulcer in mucosal edema, hemorrhage, cell desquamation, and tissue infiltration by inflammatory cells[50-52]. Then, using natural compounds, for example, polysaccharides extracted from plant seeds, is depicted as a promising alternative for protecting the gastric mucosa against the mechanism associated with peptic ulcers.
4. Conclusions
In conclusion, the galactomannan extracted from C. grandis seeds showed effective gastroprotective activity at 1.0 and 10 mg/kg concentrations. Regarding macroscopic analyses, such protection is evident for the highest concentration of galactomannan, while oxidative stress was significantly reduced in all tested concentrations, a result confirmed by the TBARS and GSH methods. Given the above, it is possible that the protection of galactomannan occurs at different levels, from the macro to the molecular and cellular one's. Regarding more specifically these molecular and cellular levels of protection, they can be corroborated by the results of the histological analysis demonstrating the extensive areas of preserved epithelium for the polysaccharide treatment in all concentrations, and also agrees with the protective potential expressed by oxidative stress results. Future works are focused on the structural basis and the exact mechanism for the gastroprotective effect of the galactomannan from C. grandis along with its digestion, absorption, and pharmacokinetic characteristics. To date, this study is essential to reinforce that the properties of molecules originating from natural products can present a great potential to be explored, especially in the biomedical area.
Acknowledgements
The authors express their gratitude to Fundação de Amparo à Ciência e Tecnologia do Estado de Pernambuco (FACEPE) and the Conselho Nacional de Desenvolvimento Científico e Tecnológico (CNPq) for financial support. T.G.S. and L.R.A.L. express their gratitude to the CNPq and FACEPE by Fellowships and Grants. J.M.A.X.B., D.M.N., and G.A.S. are grateful for the scholarship provided by the FACEPE. All authors are thankful to the Department of Morphology and Animal Physiology (UFRPE), which provided the experimental animals, and to the vivarium of the Department of Antibiotics (UFPE), where they were maintained during the entire experimental period. The authors are also grateful to the UPE Garanhuns for technical support.
Authors contribution
Barbosa JMAX, Sabiá GA: Investigation.
Nunes DM: Investigation, writing-original draft.
Soares AF: Methodology.
da Silva TG: Conceptualization.
de Lima LRA: Funding acquisition, review & editing.
Rodrigues NER: Writing-review & editing, supervision.
de Albuquerque PBS: Writing-review & editing, supervision, project administration.
Conflicts of interest
The authors declare no conflicts of interest.
Ethical approval
All experimental protocols were approved by the Ethics Committee on the Use of Animals of the Federal Rural University of Pernambuco (process number 2823030920).
Consent to participate
Not applicable.
Consent for publication
Not applicable.
Availability of data and materials
The data and materials could be obtained from the corresponding author.
Funding
The study was supported by the scholarship grant (J.M.A.C.B.) from the Fundação de Amparo à Ciência e Tecnologia de Pernambuco (FACEPE). Process number BIC-0362-2.08/20.
Copyright
© The Author(s) 2024.
References
-
1. Xu Y, Wu Y, Sun P, Zhang F, Linhardt RJ, Zhang A. Chemically modified polysaccharides: synthesis, characterization, structure activity relationships of action. Int J Biol Macromol. 2019;132:970-977.
[DOI] [PubMed] -
2. de Oliveira WF, Albuquerque PBS, dos Santos Silva PM, Coelho LCBB, dos Santos Correia MT. Gums. In: Oliveira JM, Radhouani H, Reis RL, editors. Polysaccharides of microbial origin: biomedical applications. Switzerland: Springer Nature; 2022. p. 1-35.
[DOI] -
3. Zhang H, Cui H, Xie F, Song Z, Ai L. Tamarind seeds polysaccharide: structure, properties, health benefits, modification and food applications. Food Hydrocoll. 2024;155:110222.
[DOI] -
4. Kong D, Zhang M, Mujumdar AS, Yu D. New drying technologies for animal/plant origin polysaccharide-based future food processing: Research progress, application prospects and challenges. Food Biosci. 2023;56:103315.
[DOI] -
5. Raj V, Chun KS, Lee S. State-of-the-art advancement in tara gum polysaccharide (Caesalpinia spinosa) modifications and their potential applications for drug delivery and the food industry. Carbohydr Polym. 2024;323:121440.
[DOI] -
6. Yadav S, Chamoli S, Kumar P, Maurya PK. Structural and functional insights in polysaccharides coated cerium oxide nanoparticles and their potential biomedical applications: a review. Int J Biol Macromol. 2023;246:125673.
[DOI] -
7. Wu Q, Cheng N, Fang D, Wang H, Rahman FU, Hao H, et al. Recent advances on application of polysaccharides in cosmetics. J Dermatol Sci and Cosmet Technol. 2024;1:100004.
[DOI] -
8. Chen N, Hu M, Jiang T, Xiao P, Duan JA. Insights into the molecular mechanisms, structure-activity relationships and application prospects of polysaccharides by regulating Nrf2-mediated antioxidant response. Carbohydr Polym. 2024;333:122003.
[DOI] -
9. Souza AA, Ribeiro KA, Seixas JRPC, Silva Neto JC, Santiago MGPF, Aragão-Neto AC, et al. Effects including photobiomodulation of galactomannan gel from Cassia grandis seeds in the healing process of second-degree burns. Int J Biol Macromol. 2023;251:126213.
[DOI] -
10. Chen N, Jiang T, Xu J, Xi W, Shang E, Xiao P, et al. The relationship between polysaccharide structure and its antioxidant activity needs to be systematically elucidated. Int J Biol Macromol. 2024;270(2):132391.
[DOI] -
11. Zheng P, Dai W, Tan BK, Zhang Y, Lin S, et al. Combination of steam explosion pretreatment and mixture design optimization as a strategy to enhance the antioxidant activity of crude polysaccharides isolated from edible mushrooms. LWT. 2024;200:116215.
[DOI] -
12. de Lima LRM, Silva MFS, Araújo GS, de Oliveira Silva Ribeiro F, Ribeiro IS, Pessoa C, et al. Doxorubicin-galactomannan nanoconjugates for potential cancer treatment. Carbohydr Polym. 2024;342:122356.
[DOI] -
13. Wu J, Zheng W, Luo P, Lin Z, Li F, Liang L, et al. Structural characterization of a water-soluble acidic polysaccharide CSP-IV with potential anticoagulant activity from fruit pulp of Clausena lansium (Lour.) Skeels Guifei. Int J Biol Macromol. 2024;254(3):128029.
[DOI] -
14. Sharma A, Shahid A, Banerjee R, Kumar KJ. Emerging insights into the structure-activity relationship of water-soluble polysaccharides in antiviral therapy. Eur J Med Chem Rep. 2024;10:100122.
[DOI] -
15. Tao Y, Ma J, Huang C, Lai C, Ling Z, Yong Q. The immunomodulatory activity of degradation products of Sesbania cannabina galactomannan with different molecular weights. Int J Biol Macromol. 2022;205:530-538.
[DOI] -
16. Zhang R, Zhang XZ, Guo X, Han LL, Wang BN, Zhang X, et al. The protective immunity induced by Trichinella spiralis galectin against larval challenge and the potential of galactomannan as a novel adjuvant. Res Vet Sci. 2023;165:105075.
[DOI] -
17. Dea IC, Morrison A. Chemistry and interactions of seed galactomannans. Adv Carbohydr Chem Biochem. 1975;31:241-312.
[DOI] -
18. Albuquerque PB, Barros W, Santos GR, Correia MT, Mourão PA, Teixeira JA, et al. Characterization and rheological study of the galactomannan extracted from seeds of Cassia grandis. Carbohydr Polym. 2014;104:127-134.
[DOI] -
19. Albuquerque PB, Silva CS, Soares PA, Barros W, Correia MT, Coelho LC, et al. Investigating a galactomannan gel obtained from Cassia grandis seeds as immobilizing matrix for Cramoll lectin. Int J Biol Macromol. 2016;86:454-461.
[DOI] -
20. Albuquerque PBS, Soares PAG, Aragão-Neto AC, Albuquerque GS, Silva LCN, Lima-Ribeiro MHM, et al. Healing activity evaluation of the galactomannan film obtained from Cassia grandis seeds with immobilized Cratylia mollis seed lectin. Int J Biol Macromol. 2017;102:749-757.
[DOI] -
21. de Albuquerque PBS, Rodrigues NER, Silva PMDS, de Oliveira WF, Correia MTDS, Coelho LCBB. The use of proteins, lipids, and carbohydrates in the management of wounds. Molecules. 2023;28(4):1580.
[DOI] -
22. Andrade FJET, de Albuquerque PBS, de Seixas JRPC, Feitoza GS, Barros Júnior W, Vicente AA, et al. Influence of Cassia grandis galactomannan on the properties of sponge cakes: a substitute for fat. Food Funct. 2018;9(4):2456-2468.
[DOI] -
23. Ragab TIM, Shalaby ASG, Awdan SAE, El-Bassyouni GT, Salama BM, Helmy WA, et al. Role of levan extracted from bacterial honey isolates in curing peptic ulcer: in vivo. Int J Biol Macromol. 2020;142:564-573.
[DOI] -
24. Kavitt RT, Lipowska AM, Anyane-Yeboa A, Gralnek IM. Diagnosis and treatment of peptic ulcer disease. Am J Med. 2019;132(4):447-456.
[DOI] -
25. Kuna L, Jakab J, Smolic R, Raguz-Lucic N, Vcev A, Smolic M. Peptic ulcer disease: a brief review of conventional therapy and herbal treatment options. J Clin Med. 2019;8(2):179.
[DOI] -
26. Lanas A, Chan FKL. Peptic ulcer disease. The Lancet. 2017;390(10094):613-624.
[DOI] -
27. Freedberg DE, Kim LS, Yang YX. The risks and benefits of long-term use of proton pump inhibitors: expert review and best practice advice from the american gastroenterological association. Gastroenterology. 2017;152(4):706-715.
[DOI] -
28. da Cunha Jácome Marques F, da Silva Pantoja P, Matos VEA, Silva RO, Damasceno SRB, Franco ÁX, et al. Galactomannan from the seeds of Caesalpinia pulcherrima prevents indomethacin-induced gastrointestinal damage via neutrophil migration. Int J Biol Macromol. 2019;141:68-75.
[DOI] -
29. Luo JH, Zou WS, Li J, Liu W, Huang J, Wu HW, et al. Untargeted serum and liver metabolomics analyses reveal the gastroprotective effect of polysaccharide from Evodiae fructus on ethanol-induced gastric ulcer in mice. Int J Biol Macromol. 2023;232:123481.
[DOI] -
30. de Almeida CLF, Brito SA, de Santana TI, Costa HBA, de Carvalho Júnior CHR, da Silva MV, et al. Spondias purpurea L. (Anacardiaceae): antioxidant and antiulcer activities of the leaf hexane extract. Oxid Med Cell Longev. 2017;2017:6593073.
[DOI] -
31. Ohkawa H, Ohishi N, Yagi K. Assay for lipid peroxides in animal tissues by thiobarbituric acid reaction. Anal Biochem. 1979;95(2):351-358.
[DOI] -
32. Sedlak J, Lindsay RH. Estimation of total, protein-bound, and nonprotein sulfhydryl groups in tissue with Ellman's reagent. Anal Biochem. 1968;25(1):192-205.
[DOI] -
33. Smith PK, Krohn RI, Hermanson GT, Mallia AK, Gartner FH, Provenzano MD, et al. Measurement of protein using bicinchoninic acid. Anal Biochem. 1985;150(1):76-85.
[DOI] -
34. Joshi H, Kapoor VP. Cassia grandis Linn. f. seed galactomannan: structural and crystallographical studies. Carbohydr Res. 2003;338(18):1907-1912.
[DOI] -
35. Andrade CT, Azero EG, Luciano L, Gonçalves MP. Solution properties of the galactomannans extracted from the seeds of Caesalpinia pulcherrima and Cassia javanica: comparison with locust bean gum. Int J Biol Macromol. 1999;26(2):181-185.
[DOI] -
36. Carlotto J, Maria-Ferreira D, de Souza LM, da Luz BB, Dallazen JL, de Paula Werner MF, et al. A polysaccharide fraction from "ipê-roxo" (Handroanthus heptaphyllus) leaves with gastroprotective activity. Carbohydr Polym. 2019;226:115239.
[DOI] -
37. Engels C, Gräter D, Esquivel P, Jiménez VM, Gänzle MG, Schieber A. Characterization of phenolic compounds in jocote (Spondias purpurea L.) peels by ultra highperformance liquid chromatography/electrospray ionization mass spectrometry. Food Res Int. 2012;46(2):557-562.
[DOI] -
38. Cordeiro LMC, de Fátima Reinhardt V, Baggio CH, de Paula Werner MF, Burci LM, Sassaki GL, et al. Arabinan and arabinan-rich pectic polysaccharides from quinoa (Chenopodium quinoa) seeds: Structure and gastroprotective activity. Food Chem. 2012;130:937-944.
[DOI] -
39. Tanaka LYA, de Oliveira AJB, Gonçalves JE, Cipriani TR, de Souza LM, Marques MCA, et al. An arabinogalactan with anti-ulcer protective effects isolated from Cereus peruvianus. Carbohydr Polym. 2010;82:714-721.
[DOI] -
40. da Silva BP, Parente JP. Chemical properties and antiulcerogenic activity of a galactomannoglucan from Syagrus oleracea. Food Chem. 2010;123(4):1076-1080.
[DOI] -
41. Cipriani TR, Mellinger CG, de Souza LM, Baggio CH, Freitas CS, Marques MCA, et al. Acidic heteroxylans from medicinal plants and their anti-ulcer activity. Carbohydr Polym. 2008;74(2):274-278.
[DOI] -
42. Oates PJ, Hakkinen JP. Studies on the mechanism of ethanol-induced gastric damage in rats. Gastroenterology. 1988;94(1):10-21.
[DOI] -
43. Pandian RS, Anuradha CV, Viswanathan P. Gastroprotective effect of fenugreek seeds (Trigonella foenum graecum) on experimental gastric ulcer in rats. J Ethnopharmacol. 2002;81(3):393-397.
[DOI] -
44. de Sousa Sabino LB, da Costa Gonzaga ML, de Siqueira Oliveira L, Duarte ASG, e Silva LMA, de Brito ES, et al. Polysaccharides from acerola, cashew apple, pineapple, mango and passion fruit co-products: Structure, cytotoxicity and gastroprotective effects. Bioact Carbohydr Diet Fibr. 2020;24:100228.
[DOI] -
45. Lian YZ, Lin IH, Yang YC, Chao JC. Gastroprotective effect of Lycium barbarum polysaccharides and C-phycocyanin in rats with ethanol-induced gastric ulcer. Int J Biol Macromol. 2020;165(A):1519-1528.
[DOI] -
46. Pizzorno J. Glutathione! Integr Med. 2014;13(1):8-12.
[PubMed] [PMC] -
47. Yang Q, Huang B, Li H, Zhang C, Zhang R, Huang Y, et al. Gastroprotective activities of a polysaccharide from the fruiting bodies of Pleurotus ostreatus in rats. Int J Biol Macromol. 2012;50(5):1224-1228.
[DOI] -
48. Gündogdu AC, Özbayer C, Kar F. Boric acid alleviates gastric ulcer by regulating oxidative stress and inflammation-related multiple signaling pathways. Biol Trace Elem Res. 2024;202(5):2124-2132.
[DOI] -
49. Gündogdu AC, Kar F, Özbayer C. Investigation of the gastroprotective effect of betaine-homocysteine homeostasis on oxidative stress, inflammation and apoptosis in ethanol-induced ulcer model. J Invest Surg. 2022;35(11):1806-1817.
[DOI] -
50. Simões S, Lopes R, Campos MCD, Marruz MJ, da Cruz MEM, Corvo L. Animal models of acute gastric mucosal injury: macroscopic and microscopic evaluation. Animal Model Exp Med. 2019;2(2):121-126.
[DOI] -
51. de Souza MC, Vieira AJ, Beserra FP, Pellizzon CH, Nóbrega RH, Rozza AL. Gastroprotective effect of limonene in rats: Influence on oxidative stress, inflammation and gene expression. Phytomedicine. 2019;53:37-42.
[DOI] -
52. Zhou D, Yang Q, Tian T, Chang Y, Li Y, Duan LR, et al. Gastroprotective effect of gallic acid against ethanol-induced gastric ulcer in rats: Involvement of the Nrf2/HO-1 signaling and anti-apoptosis role. Biomed Pharmacother. 2020;126:110075.
[DOI]
Copyright
© The Author(s) 2024. This is an Open Access article licensed under a Creative Commons Attribution 4.0 International License (https://creativecommons.org/licenses/by/4.0/), which permits unrestricted use, sharing, adaptation, distribution and reproduction in any medium or format, for any purpose, even commercially, as long as you give appropriate credit to the original author(s) and the source, provide a link to the Creative Commons license, and indicate if changes were made.
Publisher’s Note
Share And Cite