- 1 Department of Chemistry, Dr B R Ambedkar National Institute of Technology Jalandhar, Jalandhar 144008, Punjab, India.
- 2 Department of Chemistry, VIT-AP University, Amaravati 522241, Andhra Pradesh, India.
Sanjeeb Sutradhar, Department of Chemistry, Dr B R Ambedkar National Institute of Technology Jalandhar, Jalandhar 144008, Punjab, India. E-mail: sutradhars@nitj.ac.in
Abstract
Nanoscience and nanotechnology have steered in the new era of medical innovation and offered a promising solution to the longstanding challenges in the healthcare due to size dependent physicochemical properties. The ability to engineer the specific nanostructures makes the nanomaterials more attractive and find potential applications in the field of biomedical sciences. Among various noble metal nanomaterials, copper and copper-based nanomaterials are considered as a unique nanomaterial for multitude of the biomedical applications. Plant biomolecules contain various terpenoids, alkaloids, flavonoids, flavones, proteins, polyphenols, saponins, tannins, steroids, as well as other nutritional compounds; which are capable of acting as reducing and capping agents for copper-based nanomaterials formation. Biosynthesis of copper-based nanomaterials has great advantages due to less-toxicity, biocompatible, antiviral or anti-bacterial properties for the development of bio-sensor or drug delivery application. In this mini-review, the biosensor and drug delivery potential of the copper-based functional nano-formulation are discussed.
Keywords
1. Introduction
Copper, the 26th most abundant element in the Earth’s crust and according to man’s knowledge it is the oldest metal since 9000 B.C[1]. Copper is an essential trace element that helps to maintain homeostatic functions in living organisms. It has good electrical and thermal conductivity and has become a metal of interest due to its low toxicity, wide adaptability, higher cost effectiveness when compared to various other metals (Ag, Au, Pd, Fe, etc.) used for synthesizing of nanomaterials[2]. Copper is a co-factor for many enzymes in metabolism; yet, it can also be found in proteins that transport electrons in addition to the enzymes. There are four fundamental protein groups bonded to copper. Group I have a single tetrahedral copper ion. Group II has one copper ion with planar arrangement. Group III has two copper ions and the active site of the final group IV contains additional copper layered[3].
Copper nanomaterials are the materials synthesized in the range of 1-100 nm from vapor deposition, electrochemical reduction, radiolysis reduction, thermal decomposition, and chemical reduction of copper metal salt[4]. Classical nanomaterials synthesis processes involve the use of hazardous materials, including toxic, corrosive, and explosive substances, and utilized both chemical and physical methodologies and thus leading to harmful formation of products and waste[5]. To solve this problem, an alternative, eco-friendly, and benign method has been developed for the synthesis of clean, non-toxic nanomaterials with the help of organisms such as fungi, plants and bacteria like Bacillus subtilis, Fusarium oxysporum and Penicillium sp. as a green substitute[6].
The biosynthesized copper nanomaterials have a lot of applications; firstly, urinary tract infection (UTI) which is caused by various bacteria such as E. coli, Pseudomonas sp., Streptococcus sp., Proteus sp., Klebsiella sp., and Staphylococcus sp. majorly present in the infected area. The unique physicochemical characteristics of CuS nanoparticles are attributed to the high specific area to volume ratio. These are not only stable under harsh process conditions, but also are considered as safe materials to humans[7]. Another such application is colorectal cancer (CRC), the second most lethal cancer in both males and females. Studies have concluded that contact between nanomaterials and cell lines could lead to better response in cancer cells and reduced the concentration of the desired compound. Copper nanomaterials are highly practical because of their unique properties which induce apoptosis via reactive oxygen species (ROS) over production and DNA injury. Moreover, studies have shown that copper nanomaterials can damage the mitochondrial membrane potential and induced apoptosis through the mitochondria[8]. Another application is in the healing of chronic wounds. Copper performs a significant function in the healing activity by stimulating the expression of molecules found in the extracellular matrix, including integrin, the primary moderators of cell connection to the extracellular matrix, fibrinogen, and collagen formation. Gold and silver nanomaterials have good antibacterial properties but have some limitations. Gold nanomaterials have a limited antibacterial action against Staphylococcus aureus, Escherichia coli, Pseudomonas aeruginosa, and Candida albicans and silver nanomaterials are prone to aggregation, which might be resulted in a change in size, a loss of antibacterial activity and are poorly digested by the human body[9].
2. Biosynthesis of Copper Based Nanomaterials
Biosynthesis of nanomaterials is the synthesis of nanomaterials using plant biomolecules, microorganism, and/or enzymes. Biosynthesis of nanomaterials has gained more attention than chemical synthesis, chemical reduction method, and physical method due to its facile synthesis, economical, biocompatible, and eco-friendliness[10]. However, the success rate of nanomaterials biosynthesis depends on several factors such as survival activity, adaptability, and reducing capability of the plant biomolecules, microorganisms, and/or enzymes.
Biocompatible copper nanomaterials were synthesized with a marine bacterial species, i.e. Stenotrophomonas rhizophila strain BGNAK1 that showed significant antimicrobial activity against various pathogenic bacteria and yeast. Additionally, the scavenging ability of the copper nanomaterials against 2,2-diphenyl-1-picrylhydrazyl (DPPH), hydroxyl, nitric oxide, and superoxide radicals as well as their reducing power in the FRAP assay, demonstrated their antioxidant activity[11]. The process of producing copper nanomaterials by bacterial means, employing a strain of Bacillus flexus that was isolated from textile effluent that employed an uplift in stability of the synthesized nanomaterials. These nanomaterials were then used to clean waste-water, photocatalytically degrade different azo dyes and reduce various parameters like EC, pH, TDS, COD, color intensity, sulphates, and phosphates in the textile’s wastewater[12]. Copper nanomaterial synthesis using bacteria are copper resistant and to tackle this, a tolerant bacterial isolate (ZTB29) was also demonstrated for the synthesis of copper oxide nanomaterials[13].
The environmentally friendly production of copper oxide nanomaterials was done by utilizing flower extract from Tecoma stans (T. stans). The green nanomaterials went through agar well diffusion methods to test bactericidal efficacy against a range of clinical pathogens such as Bacillus subtilis and Pseudomonas aeruginosa bacteria. Additionally, the T. stans flower mediated copper oxide nanomaterials was shown an upper hand in degradation of methylene blue (MB) dye as well as in vitro anticancer activity against A549 lung cancer cell line[14]. Biogenically, copper nanomaterials were produced in the presence of Syzygium cumini leaf extract, that served as a great stabilizing, reducing agent, as well as showed excellent performance in terms of catalytic reduction of MB dye from the aqueous medium using sodium borohydride (NaBH4) as a reducing agent[10]. Biosynthesis of copper nanomaterials was conducted using green union strategy by ginger roots separate effectively leading to a much better antibacterial portrayal against bacteria like E. coli and S. aureus[15]. Using an easy and affordable technique, copper nanomaterials were synthesized using Curcuma longa powder aqueous extract. The Curcuma longa extract-capped copper nanomaterials showed an attractive antibacterial activity with both gram-positive and gram-negative microorganisms[16]. The potential of Ziziphus spina-christi (L.) Willd. fruit extract was investigated as novel reducing agents for the environmentally friendly manufacture of copper nanomaterials. The synthesized nanomaterials could be used as an efficient adsorptive to remove crystal violet from aqueous solution. Moreover, Escherichia coli and Staphylococcus aureus are the two distinct (gram-positive and gram-negative) bacteria, were used to test the antibacterial efficacy of the copper nanomaterials[17]. Capparis zeylanica plant leaf extract was utilized for the bio-synthesis of copper nanomaterials where the leaf extract acts as both reducing and capping agent. It showed an effective antibacterial activity more against gram negative bacteria than gram positive bacteria[18]. For the green synthesis of copper nanomaterials, Corallocarbus epigaeus rhizome extract was also used. This biogenic nanomaterials was shown effective antibacterial activity against multidrug-resistant S. typhi as well as bactericidal activity against the human pathogen, additionally it was shown a tremendous degradation efficiency for the MB[19]. Copper nanomaterials were synthesized by a green, ecofriendly method with Cissus vitiginea. The biosynthesized copper nanomaterials have strong antioxidant and antibacterial activity against DPPH free radical and UTI pathogens like E. coli, Enterococcus sp., Proteus sp. and Klebsiella sp[20]. A general schematic representation for the synthesis process used various copper metal source and various ingredients present in different leaf is shown in Figure 1.
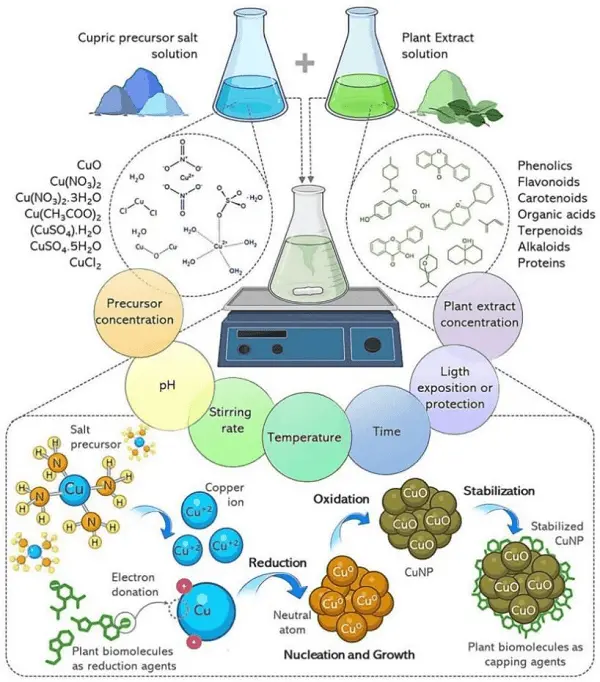
Figure 1. A schematic representation for various sources of copper, plants extract with their biomolecules present in plants extract solution, different straegy of synthesis steps and the interaction of the molecules with the core copper from copper nanomaterials[2].
3. Biosensor Application
Copper and copper-based nanomaterials can be functionalized with biologically derived recognition molecules from the plant extract solution, microorganisms, and enzymes. This allows the nanomaterials to recognize the target analyte by various spectroscopic, optical as well as electrochemical techniques. Biocompatible copper nanomaterials were synthesized using Sapindus mukorossi fruit extract. The proposed cupric oxide nanomaterials provided an eco-friendly environment for the detection of dopamine (DA) or related neurochemicals[21]. A green and simple approach was utilized for the synthesis of cuprous oxide carbon dots/Nafion (Cu2 O-CDs/NF) composite film. The addition of NF to the Cu2 O-CDs composite made the surface more homogenous due to attraction of cations via ion-exchange model and exclusion of anions (anionic uric acid, UA and ascorbic acid, AA) via electrostatic interaction. This change in the composite structure improved the analytical performance such as sensitivity and selectivity towards the detection of DA. The synthesized biogenic nanomaterials were shown a high sensitivity and reliability for detection of DA (due to oxidation of dopamine to dopamine-o-quinone, DA’) in presence of UA and AA. A schematic representation for the fabrication of electrochemical biosensor is shown in Figure 2. The nanomaterials further showed satisfactory results when applied to determine the (DA) in human serum[22]. The copper nanomaterials were added to the graphite pencil electrode (GPE) to enable enzyme-free flow injection amperometric glucose detection based on its direct oxidation in the alkaline medium without the use of any enzyme. Thus, copper nanomaterials modified GPE combination was proven to be offered fast, inexpensive, disposable, selective and sensitive amperometric sensor for the determination of glucose[23]. The study conducted and demonstrated the use of pure and copper-doped titanium dioxide (Cu-TiO2) nanostructures as the basis matrix for enzyme-less label-free myoglobin detection to detect acute myocardial infarction[24]. The creation of an electrochemical biosensor for glucose detection was done by using copper (Cu)-nanoflower-decorated gold nanomaterials (AuNMs) and graphene oxide (GO) nanofibers (NFs). When GOx-HRP-Cu nanoflower was present, Cu-nanoflower@AuNMs-GO NFs was demonstrated exceptional electrochemical catalytic nature and selectivity for the conversion of glucose to gluconic acid[25]. DA coated copper (CuO@DOP) nanomaterials were synthesized for the detection of L-cystine. The CuO@DOP nanomaterials as-developed sensor offers quick and accurate detection of L-cysteine throughout a broad concentration range as well as biocompatibility with living things[26]. A green and facile sonication method was used for the synthesis of novel maca extract coated cupric oxide nanomaterials (MaE-CuO NMs). The DA detection of the MaE-CuO NMs biosensors was evaluated on the dual electrochemical-smartphone colorimetric detection approach showcasing high sensitivity and low limit of detection value[27]. Cuprous oxide nanomaterials were synthesized by green route (P-Cu2 O NMs) with artemisia absinthium leaf extract, which acted as a reducing agent, accompanied by the enzyme tyrosinase (Tyr) used as a sensing material. P-Cu2 O+Tyr as a combination exhibited a selective response to DA and no reaction to interfering analytes such as tyrosine, cysteine, and ascorbic acid[28]. Cupric oxide nanospheres, both uncapped and glutathione-capped, were created by combining root extract of Berberis lyceum with an appropriate salt solution. GSH-CuO nanospheres were further modulated into efficient/economical biosensors[29]. Biogenically, copper oxide nanomaterials were synthesized by using Camellia japonica plant leaf extract. The synthesized nanomaterials showed effective detection of two biomolecules; namely cysteine and NADH (dihydronicotinamide adenine dinucleotide)[30].
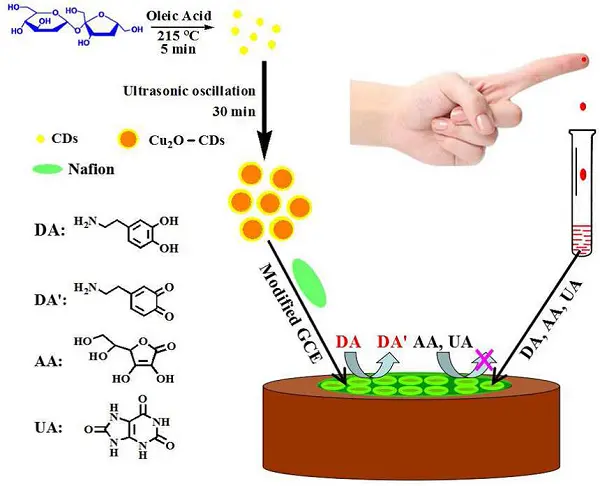
Figure 2. Biosynthesis and glassy carbon electrode fabrication strategy with Cu2 O-CDs/NF composite film for electrochemical DA detection in human serum without interference from UA and AA[22]. DA: dopamine; AA: ascorbic acid; UA: uric acid.
4. Drug Delivery Application
Biosynthesis of copper and copper-based nanomaterials has been shown as protecting therapeutic compounds, control drug release, and drug delivery to the site of disease. The copper nanomaterials were synthesized by leaf extract of the Alstonia scholaris showed antimicrobial effects on bacterial species like the S. aureus and E. coli (cf. Figure 3)[31]. The biosynthesis of copper sulphide (CuS) nanomaterials from copper sulfate was achieved with the culture supernatant of Serratia nematodiphila to show antibacterial activity against UTI pathogens such as Escherichia coli, staphylococcus aureus, proteus vulgaris and klebsiella pneumonia[7]. The green copper nanomaterials were synthesized by using Achillea millefolium leaf extracts that showed considerable antibacterial assessments against Staphylococcus aureus, M. tuberculosis, E. coli, K. pneumoniae, P. mirabili, C. diphtheriae and S. pyogenes bacteria’s as well as antifungal effects against G. albicans, A. flavus, M. canis and G. glabrata fungus[32]. Copper nanomaterials were synthesized extracellularly utilizing the stem latex of Euphorbia nivulia, a plant of medicinal importance. The nanomaterials were stabilized and subsequently capped by peptides and terpenoids present within the latex. The synthesized nanomaterials were used as a non-toxic aqueous formulations for administration of cancer therapy[33]. Biogenic copper nanomaterials were fabricated by using date stones and pomegranate peel as two waste plant extract precursors to increase its cytotoxic action. In order to assess the biogenic CuO-NMs cytotoxic potential, they were used against MCF7 human breast cancer cell lines and contrasted with the BJ1 normal cell line. The outcomes was shown a selective suppression of MCF7 cancer cell proliferation[34]. Copper oxide nanomaterials were synthesized from the leaves of (Ormocarpum cochinchinense) O. cochinchinense. The biosynthesized nanomaterials was shown a high anticancer cytotoxicity on human colon cancer cell lines (HCT-116) with IC50 value of 40 μg mL-1[35]. The copper nanomaterials were synthesized biogenically by Gloriosa superba L. plant extract. These nanomaterials exhibited significant antibacterial activity against pathogenic bacterial strains namely gram negative Klebsiella aerogenes, Pseudomonas desmolyticum, and Escherichia coli as well as gram positive bacteria Staphylococcus aureus[36]. A cost-effective one-step fast bio fabrication process for functionalized copper oxide nanomaterials was utilized the eucalyptus globulus leaf extract (ELE) bioactives. The synthesized nanomaterials were shown pro-apoptotic nature in human breast cancer (MCF-7) cells. Furthermore, ELE-CuONMs was inhibited sclerotia germination and growth of A. flavus, a type of fungal infections[37]. Copper oxide nanomaterials were synthesized by using the aqueous extract of the Stigmaphyllon ovatum plant. The biogenic nanomaterials showed effective anticancer property against the growth of the carcinoma cells, leaving less than 40% of the cells alive[38]. Biogenic copper oxide nanomaterials have shown potential larvicidal activities too. The environmentally friendly production of copper oxide nanomaterials utilizing Tridax Procumbens leaf extract was investigated larvicidal activity against dengue, chikungunya, and zika, causing vector Aedes aegypti. Copper oxide nanomaterials was exhibited the highest level of activity with the Aedes aegypti[39]. A comparative performance of various bio-based copper nanomaterials in drug delivery is shown in Table 1.
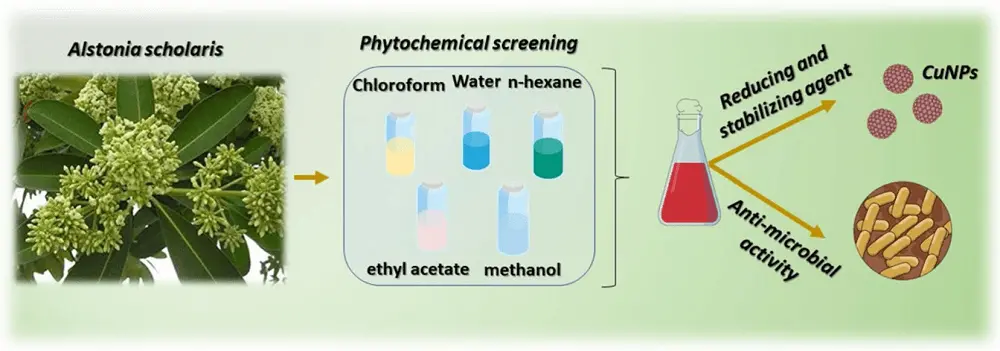
Figure 3. A general aim for the investigation of anti-microbial activity based on copper nanomaterials[31].
Nanomaterials | Sources for biosynthesis | Applications | References |
Cu-NMs | Alstonia scholaris leaf extract | Antimicrobial effects on bacterial species like the S. aureus and E. coli | [30] |
CuO NMs | Achillea millefolium leaf extract | Antibacterial and anti-fungal effects on Staphylococcus aureus, M. tuberculosis, E. coli, K. pneumoniae, P. mirabili, C. diphtheriae and S. pyogenes bacteria’s and G. albicans, A. flavus, M. canis and G. glabrata | [31] |
Cu-NMs | Euphorbia nivulia latex | Used for administration/in vivo delivery of nanomaterials for cancer therapy | [32] |
CuO-NMs | Pomegranate peel and date stones plant extract | Cytotoxic agent against human breast cancer [MCF7] cell lines | [33] |
CuO-NMs | Ormocarpum cochinchinense leaf extract | Anticancer cytotoxicity with IC50 value of 40 μg/mL on human colon cancer cell lines (HCT-116) | [34] |
CuO-NMs | Eucalyptus globulus leaf extract | Apoptotic effect on human breast cancerMCF-7 cells with a significant (P <0.05) reduction in viability and inhibited sclerotia germination and growth of A. flavus | [36] |
CuO-NMs | Stigmaphyllon ovatum leaf extract | Anticancer agents in HeLa cells with IC50 value of 40μM | [37] |
CuO-NMs | Tridax procumbens leaf extract | Larvicidal activity against chikungunya, yellow fever, and dengue transmit vector Aedes aegypti species | [38] |
5. Conclusions and Future Perspectives
The large number of medicinal plants and microorganisms can be used for fabrication and functionalization of copper and copper-based nanomaterials due to the availability of various biomolecules in them. This enhanced to achieve the aim of green, eco-friendly, and one-step route in view of the environmental consequences. The development of different methodologies to extract of phytocompounds would be allowed to improve the stabilize of nanomaterials and preserved their physical as well as chemical properties for longer period. To interpret this, it would be necessary to understand the biochemical component composition or structure and the molecular level mechanism for the formation of nanomaterials. Bio-mediated synthesis of copper or copper-based nanomaterials has been applied to treat many pathogenic/carcinogenic diseases with lower toxic effect. However, non-bio-mediated synthesis of copper or copper-based nanomaterials has various toxic effects for the human health. It is an obvious challenge to identify and isolate the bio-component from the plant or its extract, microorganisms and/or enzymes that contribute to the nano-formulation and stabilization. This presents an opportunity to explore the bio-based advanced functional materials.
Acknowledgments
Chattopadhyay S, Kataria S, Prusty S, Subban M, Sutradhar S acknowledge the Department of Chemistry, Dr. B R Ambedkar National Institute of Technology Jalandhar for providing support and facilities. Prusty S acknowledges to Department of Chemistry, Vellore Institute of Technology, Andhra Pradesh, for support and facilities.
Authors contribution
Chattopadhyay S, Sutradhar S, Prusty S: Literature collection and analysis, writing manuscript, editing and final decision.
Kumar A, Subban M: Reference writing, writing manuscript, editing and final decision.
Kataria S: Writing manuscript, editing and final decision.
Conflicts of interest
The authors declare no conflicts of interest.
Ethical approval
Not applicable.
Consent to Participate
Not applicable.
Consent for Publication
Not applicable.
Funding
This work was supported by the Dr B R Ambedkar National Institute of Technology Jalandhar for funding through seed grant (NITJ/DRC/4710) and the Vellore Institute of Technology, Andhra Pradesh, for funding through the RGEMS scheme (VIT-AP/SPORIC/RGEMS/2022-23/002).
Copyright
© The Author(s) 2024.
References
-
1. Mitra D, Kang ET, Neoh KG. Antimicrobial copper-based materials and coatings: potential multifaceted biomedical applications. ACS Appl Mater Interfaces. 2020;12(19):21159-21182.
[DOI] -
2. Antonio-Perez A, Duran-Armenta LF, Perez-Loredo MG, Torres-Huerta AL. Biosynthesis of copper nanoparticles with medicinal plants extracts: From extraction methods to applications. Micromachines. 2023;14(10):1882-1882.
[DOI] -
3. Pohanka M. Copper and copper nanoparticles toxicity and their impact on basic functions in the body. Bratisl Med J. 2019;120(6):397-409.
[DOI] -
4. Thenmozhi M, Manimegalai V. Green synthesis of copper oxid nanoparticles using Allmania nodiflora leaf extract and its characterization. BME Horiz. 2023;1(2):69-69.
[DOI] -
5. Jamkhande PG, Ghule NW, Bamer AH, Kalaskar MG. Metal nanoparticles synthesis: An overview on methods of preparation, advantages and disadvantages, and applications. J Drug Del Sci Tech. 2019;53:101174-101174.
[DOI] -
6. Jadoun S, Arif R, Jangid NK, Meena RK. Green synthesis of nanoparticles using plant extracts: A review. Environ Chem Lett. 2020;19(1):355-374.
[DOI] -
7. Chelladurai M, Shanmugam R. Invitro bactericidal activity of bio-synthesized CuS nanoparticles against UTI causing pathogens. Inorg Nano-Met Chem. 2016;47(9):1290-1297.
[DOI] -
8. Ghasemi P, Shafiee G, Ziamajidi N, Abbasalipourkabir R. Copper Nanoparticles Induce Apoptosis and Oxidative Stress in SW480 Human Colon Cancer Cell Line. Biol Trace Elem Res. 2023;201(8):3746-3754.
[DOI] -
9. Sandoval C, Rios G, Sepulveda N, Salvo J, Souza-Mello V, Farias J. Effectiveness of copper nanoparticles in wound healing process using in vivo and in vitro studies: A systematic review. Pharmaceutics. 2022;14(9):1838-1838.
[DOI] -
10. Din MI, Akbar R, Hussain Z, Khalid R, Arshad M, Hussain T, Khan SA. Biosynthesis of monodispersed stable copper nanoparticles using Syzygium cumini: characterization and potential applications. Desalin Water Treat. 2023;281:130-136.
[DOI] -
11. Karthikeyan A, Gopinath N, Nair BG. Ecofriendly biosynthesis of copper nanoparticles from novel marine S. rhizophila species for enhanced antibiofilm, antimicrobial and antioxidant potential. Microb Pathog. 2024;194:106836-106836.
[DOI] -
12. Batool F, Shahid M, Mahmood F, Shahzad T, Azeem F, Hussain S, et al. Biosynthesis of copper nanoparticles using Bacillus flexus and estimation of their potential for decolorization of azo dyes and textile wastewater treatment. J King Saud Univ.Sci. 2024;36(8):103309.
[DOI] -
13. Singh D, Jain D, Rajpurohit D, Jat G, Kushwaha HS, Singh A, et al. Bacteria assisted green synthesis of copper oxide nanoparticles and their potential applications as antimicrobial agents and plant growth stimulants. Front Chem. 2023;11:1154128-1154128.
[DOI] -
14. Vasantharaj S, Sathiyavimal S, Bharathi D, Pannerselvam B, Jeon S, Srituravanich W. Biosynthesis of copper oxide nanoparticles using Tecoma stans flower extract and its antibacterial, anticancer, and photocatalytic activities. Biocatal Agric Biotechnol. 2024;58:103137-103137.
[DOI] -
15. Abbas AH, Fairouz NY. Characterization, biosynthesis of copper nanoparticles using ginger roots extract and investigation of its antibacterial activity. Mater Today Proc. 2022;61:908-913.
[DOI] -
16. Jayarambabu N, Akshaykranth A, Rao TV, Rao KV, Kumar RR. Green synthesis of Cu nanoparticles using Curcuma longa extract and their application in antimicrobial activity. Mater Lett. 2020;259:126813-126813.
[DOI] -
17. Khani R, Roostaei B, Bagherzade G, Moudi M. Green synthesis of copper nanoparticles by fruit extract of Ziziphus spina-christi (L.) Willd.: Application for adsorption of triphenylmethane dye and antibacterial assay. J Mol Liq. 2018;255:541-549.
[DOI] -
18. Saranyaadevi K, Subha V, Ravindran RE, Renganathan S. Synthesis and characterization of copper nanoparticle using capparis zeylanica leaf extract. Int J Chemtech Res. 2014;6(10):4533-4541. Available from: https://sphinxsai.com/2014/race/2/(4533-4541)%20014.pdf
-
19. Selvam K, Sudhakar C, Selvankumar T, Senthilkumar B, Selva Kumar, Kannan N. Biomimetic synthesis of copper nanoparticles using rhizome extract of Corallocarbus epigaeus and their bactericidal with photocatalytic activity. SN Appl Sci. 2020;2(6):1028-1028.
[DOI] -
20. Wu S, Rajeshkumar S, Madasamy M, Mahendran V. Green synthesis of copper nanoparticles using Cissus vitiginea and its antioxidant and antibacterial activity against urinary tract infection pathogens. Artif Cells Nanomed Biotechnol. 2020;48(1):1153-1158.
[DOI] -
21. Sundar S, Venkatachalam G, Kwon SJ. Biosynthesis of copper oxide (CuO) nanowires and their use for the electrochemical sensing of dopamine. Nanomaterials. 2018;8(10):823-823.
[DOI] -
22. Huang Q, Lin X, Lin C, Zhang Y, Hu S, Wei C. A high performance electrochemical biosensor based on Cu2O-carbon dots for selective and sensitive determination of dopamine in human serum. RSC Adv. 2015;5(67):54102-54108.
[DOI] -
23. Ayaz S, Karakaya S, Emir G, Dilgin DG, Dilgin Y. A novel enzyme-free FI-amperometric glucose biosensor at Cu nanoparticles modified graphite pencil electrode. Microchem J. 2020;154:104586-104586.
[DOI] -
24. Umar A, Haque M, Ansari SG, Seo HK, Ibrahim AA, Alhamami MA, et al. Label-free myoglobin biosensor based on pure and copper-doped titanium dioxide nanomaterials. Biosensors. 2022;12(12):1151-1151.
[DOI] -
25. Baek SH, Roh J, Park CY, Kim MW, Shi R, Kailasa SK, Park TJ. Cu-nanoflower decorated gold nanoparticles-graphene oxide nanofiber as electrochemical biosensor for glucose detection. Mater Sci Eng C. 2020;107:110273-110273.
[DOI] -
26. Rohilla D, Chaudhary S, Kaur N, Shanavas A. Dopamine functionalized CuO nanoparticles: A high valued "turn on" colorimetric biosensor for detecting cysteine in human serum and urine samples. Mater Sci Eng C. 2020;110:110724-110724.
[DOI] -
27. Ilgar M, Baytemir G, Tasaltin N, Gullulu S, Yesilyurt IS, Karakus S. Multifunctional maca extract coated CuO nanoparticles with antimicrobial and dopamine sensing activities: A dual electrochemical - Smartphone colorimetric detection system. J Photochem Photobiol A. 2022;431:114075-114075.
[DOI] -
28. Pavithra N, Johri S, Varshney R, Ramamurthy PC. Dopamine fluorescent sensor based on green synthesized copper oxide nanoparticles and tyrosinase. J-Flex. 2023;2(2):183-189.
[DOI] -
29. Ain NU, Safdar N, Yasmin A. Additive-based stability assessment of biologically designed CuO and GSH-CuO nanospheres and their applicability as Nano-biosensors. Colloids Surf B Biointerfaces. 2019;178:66-73.
[DOI] -
30. Muthuchamy M, Muneeswaran T, Rajivgandhi G, Franck Q, Muthusamy A, Ji-Ming S. Biologically synthesized copper and zinc oxide nanoparticles for important biomolecules detection and antimicrobial applications. Mater Today Commun. 2020;22:100766-100766.
[DOI] -
31. Labaran AN, Zango ZU, Tailor G, Alsadig A, Usman F, Mukhtar MT, et al. Biosynthesis of copper nanoparticles using Alstonia scholaris leaves and its antimicrobial studies. Sci Rep. 2024;14(1):5589-5589.
[DOI] -
32. Rabiee N, Bagherzadeh M, Kiani M, Ghadiri AM, Etessamifar F, Jaberizadeh AH, Shakeri A. Biosynthesis of copper oxide nanoparticles with potential biomedical applications. Int J Nanomedicine. 2020;15:3983-3999.
[DOI] -
33. Valodkar M, Jadeja RN, Thounaojam MC, Devkar RV, Thakore S. Biocompatible synthesis of peptide capped copper nanoparticles and their biological effect on tumor cells. Mater Chem Phys. 2011;128(1):83-89.
[DOI] -
34. Mahmoud NM, Mohamed HI, Ahmed SB, Akhtar S. Efficient biosynthesis of CuO nanoparticles with potential cytotoxic activity. Chem Pap. 2020;74(9):2825-2835.
[DOI] -
35. Gnanavel V, Palanichamy V, Roopan SM. Biosynthesis and characterization of copper oxide nanoparticles and its anticancer activity on human colon cancer cell lines (HCT-116). J Photochem Photobiol B. 2017;171:133-138.
[DOI] -
36. Naika HR, Lingaraju K, Manjunath K, Kumar D, Nagaraju G, Suresh D, Nagabhushana H. Green synthesis of CuO nanoparticles using Gloriosa superba L. extract and their antibacterial activity. J Taibah Univ Sci. 2015;9(1):7-12.
[DOI] -
37. Ali K, Saquib Q, Ahmed B, Siddiqui MA, Ahmad J, Al-Shaeri M, et al. Bio-functionalized CuO nanoparticles induced apoptotic activities in human breast carcinoma cells and toxicity against Aspergillus flavus: An in vitro approach. Process Biochem. 2020;91:387-397.
[DOI] -
38. Elemike EE, Onwudiwe DC, Nundkumar N, Singh M. CuO and Au-CuO nanoparticles mediated by Stigmaphyllon ovatum leaf extract and their anticancer potential. Inorg Chem Commun. 2019;104:93-97.
[DOI] -
39. Muthamil Selvan, Vijai Anand, Govindaraju K, Tamilselvan S, Kumar VG, Subramanian KS, et al. Green synthesis of copper oxide nanoparticles and mosquito larvicidal activity against dengue, zika and chikungunya causing vector Aedes aegypti. IET Nanobiotechnol. 2018;12(8):1042-1046.
[DOI]
Copyright
© The Author(s) 2024. This is an Open Access article licensed under a Creative Commons Attribution 4.0 International License (https://creativecommons.org/licenses/by/4.0/), which permits unrestricted use, sharing, adaptation, distribution and reproduction in any medium or format, for any purpose, even commercially, as long as you give appropriate credit to the original author(s) and the source, provide a link to the Creative Commons license, and indicate if changes were made.
Share And Cite