Abstract
Insufficiency and near absence of green infrastructure (GI) which have been shown to offer cost-effective nature-based solutions in mitigating urban flood and managing stormwater in major cities in Nigeria have increased their vulnerability to destructive floods in recent years. This study conducted a spatial assessment of GI and its potential effectiveness on stormwater management in Ibadan, Nigeria, with the use of remotely sensed data. Change detection between 2000 and 2020 was conducted based on four land use categories (built-up, green area, bare surface, and water bodies) because it coincided the period of rapid land use changes and occurrence of devastating flooding in the city and environs. Normalized Difference Vegetative Index (NDVI) calculated from Landsat image data and Digital Elevation Model (DEM) data to determine areas liable to flooding were also assessed using geospatial analysis. The results showed that the infill development of Ibadan has drastically reduced the available GI from 37.64% in the year 2000 to 22.84% in the year 2020 while built-up areas increased from 51.97% in the year 2000 to 69.04% in the year 2020. In 2000, NDVI ranged from -0.37 to 0.02 in the inner city showing poor vegetation. By 2020 the elements of GI are mostly fragmented with small coverage and more than 70% of the area occupied by built-up areas without vegetation cover. Vegetal cover decreased with time due to increasing impervious surfaces, creating many high flood zones and stormwater. It is concluded that provision and effective management of GI in the city can serve as a valuable tool to mitigate the problems of excessive stormwater.
Keywords
1. Introduction
In recent decades, rapid population growth and urbanization have become major challenge to urban planner, environmentalists and other stakeholders. More so, the urban population is expected to increase to 68% of the world's population by 2050[1]. The continuous growth of the urban has increased demand for more space in the expanding urbanized areas, which has often brought about negative consequences in natural environments. Rapid urbanization has led to the replacement of existing natural greenspaces with impervious surfaces[2] that affects the function of watersheds directly or indirectly by changing the rate/volume of surface runoff and the frequency/severity of storm events[3]. Most urban areas consist of impervious surfaces such as asphalt, concrete, metals and other materials which are made of gray or durable infrastructures that prevent stormwater from soaking directly into the soil[4,5]. In the event of a storm in such areas, the accumulation of rainfall can distribute debris and pollutants along its path, as well as cause flooding or blockages in drainage channels.
Anthropogenic activities such as the conversion of natural, agricultural and other lands into various physical infrastructural projects has inevitably changed the hydrology of the various settlements[6,7]. Many cities have grown exponentially with the reduction in vegetative land cover creating difficult stormwater management[8-12] Green infrastructures (GI) which are a variety of water management practices such as roadsides planting, vegetated rooftops, and absorbent gardens that helps to prevent polluted stormwater and runoff from reaching sewers, streams, rivers, and larger water bodies are generally absent or insufficient in the urban areas[13-16]. Stormwater is the surplus water that accumulates on the ground during heavy rainfall. This water gathers pollutants such as trash, chemicals, and sediment as it flows through streets and other surfaces ultimately finds its way into water bodies, compromising water quality and posing a significant threat to public health[12,17-19].
Studies have shown that GI is a spatial structure that provides numerous services that are considered to be an effective approach to reducing the adverse impacts of urbanization on hydrological cycle[20,21]. GI is a promising on-site nature-based stormwater management method that contribute to reversing hydrological and water quality impacts of urbanization by reducing the volume and speed of stormwater[16,22-24]. Although, there is a lack of understanding of the impacts of underlying spatial patterns of GI on the enhancement of resilience[24-26] the implementation and expansion of GI has been recognized as a good strategy to build resilience into urban drainage systems and stormwater management[14,24,27,28]. Major cities especially in developing countries do not have stormwater green infrastructure (SWGI) as part of their physical development agenda and this has hindered the growth of the greenspaces which are been lost to urban sprawl and other physical infrastructural development[29,30]. The development of GI for stormwater management is one of the approaches suggested to improve the resilience of cities[11,31], because they are networks of natural and semi-natural green spaces designed to infiltrate and treat surface runoff and also mitigate the effects of climate change[32-34].
Weak land use planning systems have remained feasible the bane of urban development in Nigerian cities leading to unplanned and haphazard growth[35]. Ibadan is a major city in the southwestern part of the country that is bedeviled with the ugly fate of uncontrolled and unplanned growth[36] as it is growing faster at astronomical rate[37]. This has resulted in the drastic reduction in vegetative land cover causing a number of environmental impacts such as flooding, air and water pollution[38]. Available records show that Ibadan has no proper master plan[39,40] which brought about lack of planning, preservation and maintenance of green infrastructure in the city for effective storm water management. In August 2011, a devastating flood disaster was experienced in the city that caused a lot of woes to people around Apete, Idi-Ishin, Apata, Odo-Ona Elewe, Oke-Ayo, Ologuneru, and University of Ibadan. It was reported that 506 people lost their lives and material loss was estimated to be around 190 million naira[41]. The degradation of the Eleyele wetlands is seen as a major cause, compounded by anthropogenic impacts (indiscriminate dumping of refuse, encroachment upon wetlands, and poor channelization of drainages) and the emerging reality of climate change[42,43]. Major rivers like Ogunpa, Kudeti, and Orogun rivers are often used as dumping site for municipal solid wastes. Given the fact that GI can help contribute to resilience[17], there is the need to design and formulate strategies for stormwater management using a powerful and effective tool such as the geospatial information systems[5,7.44,45]. According to Conley et al. [5], there are many co-benefits of what is called green stormwater infrastructure that are related to increased vegetative cover. This can be measured with satellite imagery via spectral indices such as the Normalized Difference Vegetation Index (NDVI) which has not been given any thought by the various tiers of governments and agencies in the city. Remote sensing effectively collects multi-temporal, multi-spectral and multi-location data to assist in observing land use changes while geographic information system provides a spatial platform for analyzing and displaying digital data and acts as a decision support system[46]. This paper therefore sought the application of geospatial technologies to have insight into the green infrastructure of Ibadan through data collection, analysis and evaluation with a view to exploring the benefits to be accrued and improving resilience of the city in storm water management.
2. Methodology
2.1 Study area
Ibadan metropolis (Figure 1) is one of the largest and fast-growing cities in Nigeria. It is located on latitude 7°25' to 7°43' N and longitude 3°62 to 3°95 E and approximately 145 km North of Lagos (the commercial hub of the country). It is a highly built-up with dense population which is currently estimated to be about 4 million inhabitants[47]. It lies above an elevation of 227 m above sea level[48] with a very intensive system of rivers and streams. The two major rivers are River Ogunpa and River Kudeti, while others are River Gege and River Olodo. The metropolis has five local government areas (Ibadan North, Ibadan South, Ibadan North West, Ibadan South-West and Ibadan North East). River Ogunpa which bisects the core of the city was canalized due to reoccurrence of flood and high stormwater level after high rainfalls. However, anthropogenic activities such as loss of greenspaces to uncontrolled developments and encroachment of the floodplains have resulted in huge amount of overland flow and flooding in major parts of the metropolis.
2.2 Data acquisition
Satellite images for 2000, 2005, 2010, 2015 and 2020 acquired from Landsat archives of United States Geological Surveys (USGS) covering 20 years were used. The supervised classification method was adopted in the study using the maximum likelihood algorithm[49]. Five cloud-free images covering the study period were downloaded. The images were projected using WGS-1984 Zone 31. The 2020 image was used as the base image for making automatic registrations of other images with root mean square errors (RMSE) less than 0.5 pixels. These images were then clipped to the geographic boundary of the study area to create the five final images during study periods. Primary data such as the location of the GI was also collected using handheld Global Positioning System (GPS) to capture its location coordinates (x, y) with reference to a datum. Table 1 described the characteristics of the Landsat resources used.
S/No. | Sensor | Date of Acquisition | Path/Row | Band combination used | Resolution (m) | Format | Source |
1 | Landsat 5 TM | December 17, 2000 | 191/055 | 1,2,3,4,5, 6 and 7 | 30 m | GeoTIFF | USGS |
2 | Landsat 5 TM | December 18, 2005 | 191/055 | 1,2,3,4,5, 6 and 7 | 30 m | GeoTIFF | USGS |
3 | Landsat 7 TM | January 01, 2010 | 191/055 | 1,2,3,4,5, 6 and 7 | 30 m | GeoTIFF | USGS |
4 | Landsat8 OLI_TIR | January 06, 2015 | 191/055 | 1,2,3,4,5, 6 and 7 | 30 m | GeoTIFF | USGS |
5 | Landsat8 OLI_TIR | January 04, 2020 | 191/055 | 2,3,4,5, 6,7, 10 and 11 | 30 m | GeoTIFF | USGS |
TM: thematic mapper; OLI: operational land imager.
2.3 Image pre-processing
For this study, images from the Landsat for 2000, 2005, 2010, 2015 and 2020, were obtained from the USGS database. All of the bands used have a spatial resolution of 30m. The images were corrected for radiometric and geometric errors, and spectral bands were layer stacked to create composite images of the study area for each year. The Landsat data sets were pre-referenced with Universal Transverse Mercator projection system and ArcGIS 10.5 and SPSS 22 software were used for land use/land cover (LULC) analysis.
2.4 Classification method
The supervised image classification was carried out with maximum likelihood method to show the changes of land use/land cover over time. The acquired satellite images were classified into four land use and land cover (LULC) classes: built-up, green area, bare surface, and water bodies. Furthermore ground-truthing was carried out in order to gain prior knowledge of the study area and to select training areas. The training areas were selected and the Landsat images classified using the maximum-likelihood algorithm[49]. Visual image interpretation was performed using field knowledge and Google Earth images of the study area. The spectral information contained in individual pixels is used to generate LULC classes and final land cover maps for different years. Classification accuracy was evaluated, and the Kappa coefficients ranged from 85.3% to 94.5%. Transition matrix was created in ArcGIS 10.5 using cross tabulation to analyze changes in land use/ land cover in the study area from 2000 to 2020. For this research, image differencing was conducted to determine changes between images of different periods. The difference between two images is calculated by subtracting the imagery of one date from that of another, and generating an image based on the result. Percentage change to determine the trend of change can then be calculated by dividing observed change by sum of changes multiply by 100
In addition, urban green infrastructure was measured by the NDVI based on the reflective properties of leaves in red and near infrared wave length[50,51]. NDVI measurements can be used to quantify urban greenness changes and provide a proxy measurement to track their vegetation-related or co-benefits[4]. The NDVI scale runs from -1 to 1 and the values are positive for vegetation such as crops, shrubs, grasses, and forests[51-53], while and near zero or negative for non-vegetated areas such as built-up areas, concrete surfaces, rocks, bare sand and water body[54]. It is calculated using the equations below:
where NDVI = normalized difference vegetation index, ρNIR is the surface reflectance of band 4, and ρRed is the surface reflectance of band 3 in Landsat 5 and 7, and band 5 and band 4 in Landsat 8).
NDVI time series was created using Tier 1 Surface Reflectance data from Landsat 5 Thematic Mapper (TM), Landsat 7 Enhanced Thematic Mapper Plus (ETM+), and Landsat 8 Operational Land Imager (OLI) sensors for years 2000-2005, 2010, and 2015-2020, respectively. The NDVI maps were reclassified into three categories namely: low, moderate and high. NDVI was calculated for three periods: 2000, 2010 and 2020 to coincide with the periods of flooding in the study area.
The SRTM DEM data downloaded from the United States Geological Survey (USGS) was used to perform some hydrologic analysis in ArcGIS for the study area. Various maps, including the fill, flow direction, flow accumulation, stream order, drainage networks, slope and aspect were generated to prepare the flood zone maps. The maps were generated using hydrology tool in the raster calculator in ArcMap 10.5. These were then used to conduct flood vulnerability in the study area the possible flood-prone locations in the study area. The resultant flood maps were categorized into four flood vulnerability groups namely: low, moderate, high and very high. These maps were then overlay on the reclassified NDVI maps to check the relationships between the presence of vegetation (GI) and the vulnerability to flood or high amount of stormwater.
3. Results and Discussion
3.1 Land use/land cover analysis
The predominant land uses in the area are categorized into four classes: built-up area, green area, bare surface and water body. The results of the analysis show a gradual increase in the built-up areas at the detriment of the green areas which represents the green infrastructures (Table 2 and Figure 2). In year 2000, built-up area covered about 70.67 km2 (51.97%) while the green area was about 51.17 km2 (37.64%). However due to continuous physical development and rapid population growth, more green areas were lost to built-up and by 2020, the built-up area had increased to 93.87 km2 (69.04%) while the green area reduced drastically to 31.07 km2 (22.84%).
YearLULC | 2000 | 2005 | 2010 | 2015 | 2020 | |||||
Sqkm | % | Sqkm | % | Sqkm | % | Sqkm | % | Sqkm | % | |
Built-up | 70.66 | 51.97 | 75.85 | 55.79 | 77.50 | 57.00 | 91.27 | 67.13 | 93.87 | 69.04 |
Green Area | 51.17 | 37.64 | 47.53 | 34.97 | 47.99 | 35.30 | 31.65 | 21.96 | 31.07 | 22.84 |
Bare surface | 13.64 | 10.04 | 12.15 | 8.94 | 12.426 | 9.13 | 12.84 | 10.69 | 10.90 | 8.00 |
Water bodies | 0.48 | 0.36 | 0.42 | 0.31 | 0.40 | 0.30 | 0.30 | 0.22 | 0.12 | 0.12 |
Total | 135.96 | 100.00 | 135.96 | 100.00 | 135.96 | 100.00 | 135.96 | 100.00 | 135.96 | 100.00 |
LULC: land use and land cover.
The increasing demands for land for housing, commercial activities, industrial expansion, and transportation were the major drivers of the continuous loss of the vegetal covers[55]. Land use statistics in Ibadan from year 2000 to 2020 revealed that more than 50% of the land had been converted to built-up due to rapid increase in the growth of the urban areas. This is accompanied by spatial increase in the impervious surfaces, which has direct impact on hydrological cycle of watershed by preventing surface flow and infiltration.
This result confirmed the findings of that conversion of vegetative cover into impervious cover leads to hydrological, physicochemical and biological disturbances of the watershed[56,6] also observed that substantial expansion of urban features and unsystematic urban growth and unplanned urbanization threatens the sustainability of the development process, which affect critical environmental components.
Widespread expansion of impervious surfaces in an urban area like Ibadan metropolis increases in stormwater runoff has detrimental impacts on water bodies, ranging from hydrologic regime alteration, erosion, and increased pollutant transport and loading[2,5]. Thus, protecting as well as maintaining healthy green spaces will be an important matter for the future development
3.2 NDVI mapping
The NDVI of the study areas revealed the categories of vegetation. In years 2000, 2010 and 2020 in Ibadan, vegetation index varies from -0.3704 to 0.0151, 0.0075 to 0.3694, and 0.0273 to 0.2593 respectively (Figure 3). It shows a gradual decline in the vegetation in the study areas due mostly to anthropogenic or natural factors. The indices decreased with progression of the years showing preponderant devaluation of green spaces.
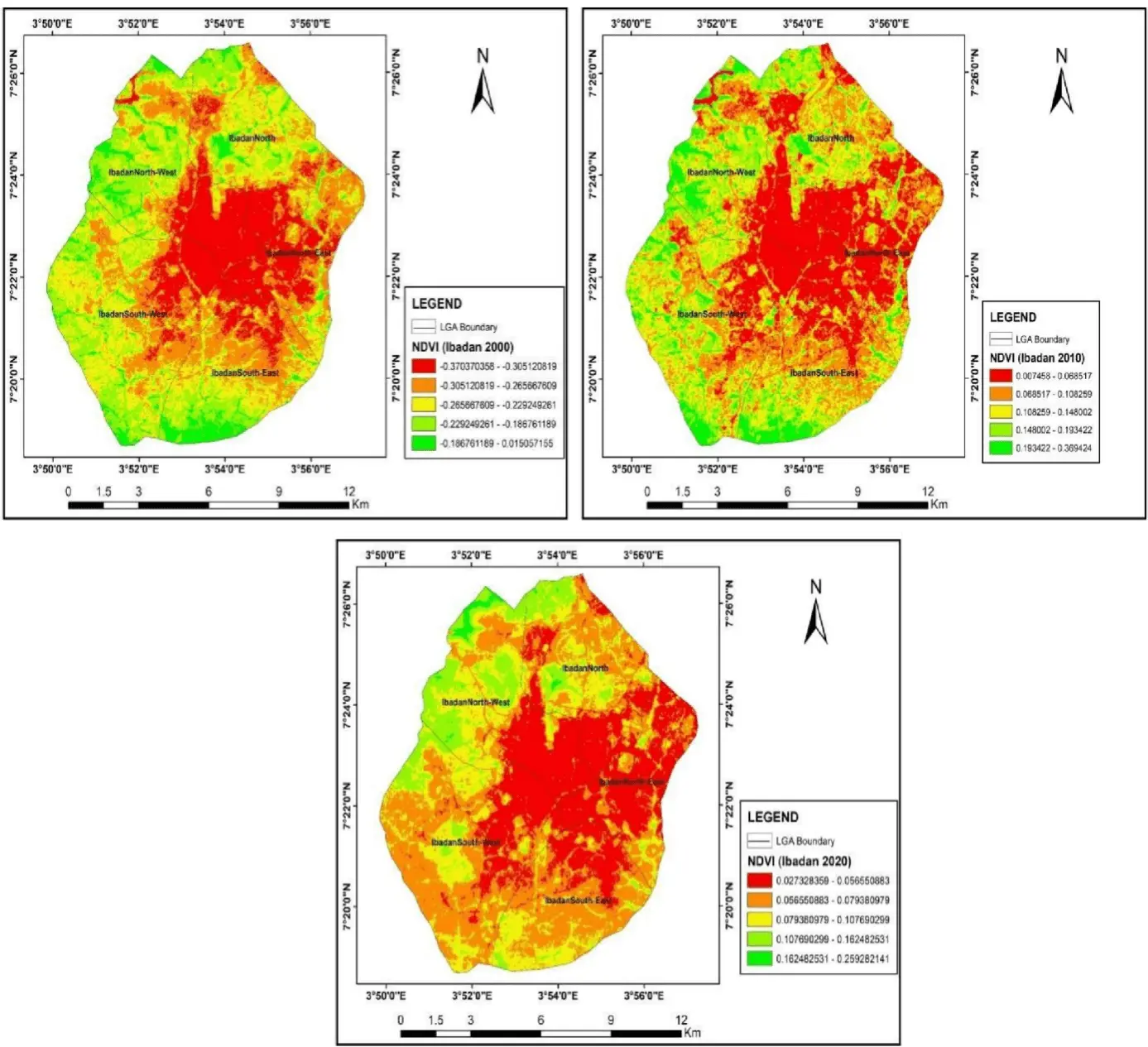
Figure 3. NDVI in Ibadan (2000, 2010 and 2020). NDVI: Normalized Difference Vegetation Index.
The negative NDVI trends can be linked to the expansion of the urban footprints within the study area over time[5]. The NDVI change detection map showed vegetation loss in the major parts of the study area.
3.3 Flood vulnerability
The SRTM DEM data was used to perform some hydrologic analysis in ArcGIS for the study area and the result was presented in raster format to indicate zones that are susceptible to flooding. The result displayed areas of low flooding, moderate flooding, and high flooding. Figure 4 shows the different maps generated to identify the flood-prone areas. Generally, flow accumulation indicates the degree of surface flow concentration and the values often increase in the downstream as concentration of flow increases[57]. According to flow accumulation highlights the amount of flow accumulated in each raster pixel based on the cumulative weights of the preceding pixels[58,59]. It is revealed that the downstream areas are more prone to flood because their flow accumulation is very high. It was revealed that areas with high slopes and flood zones corresponded with those built-up areas with several impervious surfaces and little or no vegetation (GI). These areas are not covered with good vegetation that could prevent excessive water accumulation.
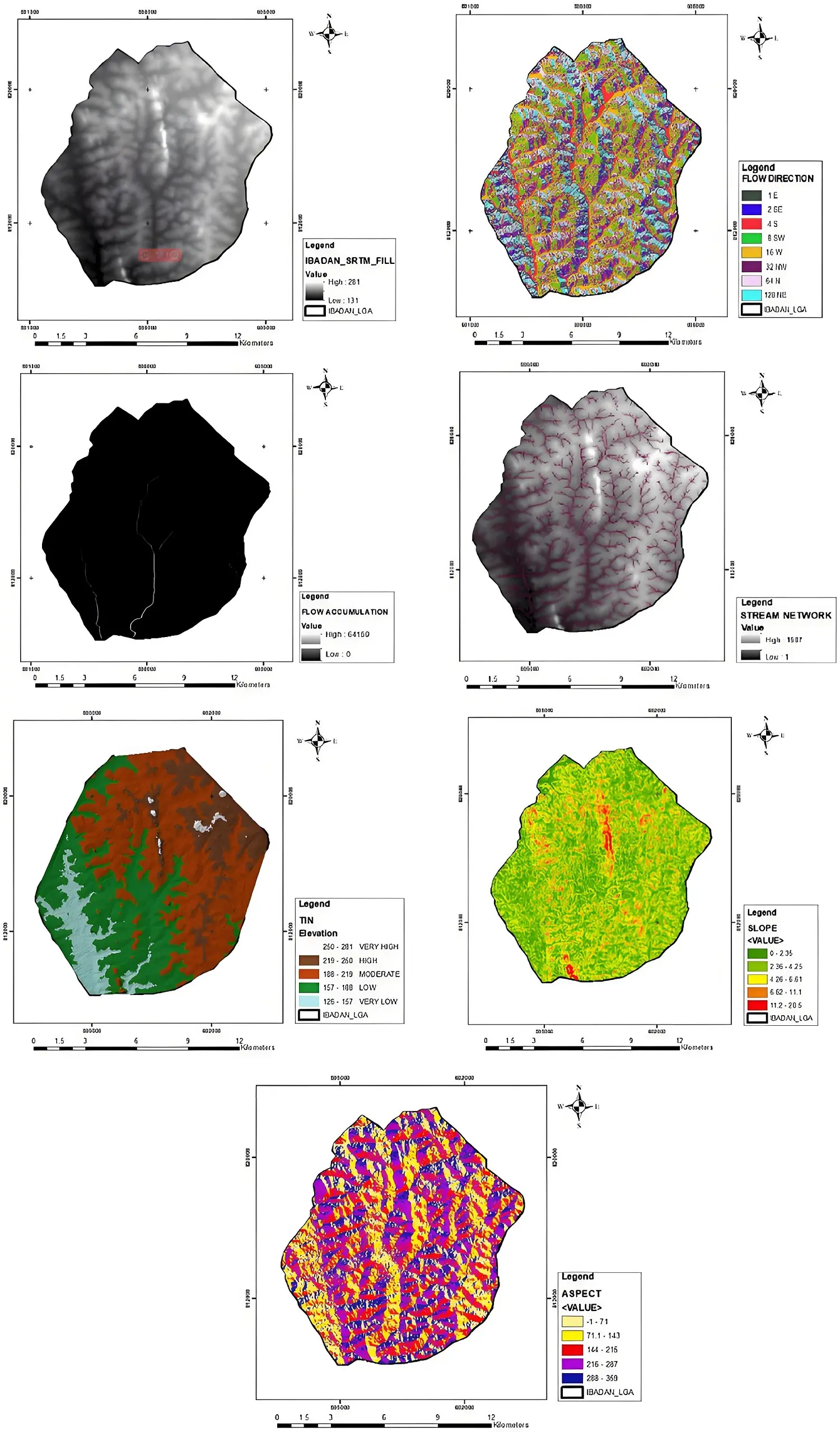
Figure 4. Fill, flow direction, flow accumulation, drainage networks, elevation, slope and aspect.
Furthermore, north central parts of the study area are dominated by low elevation, lower slope, high drainage density and flow accumulation making them highly susceptible to flood. Slope controls the velocity of overland flow and the concentration of flow hence places with high slopes drain water quickly, while those with low slopes may lead to the stagnation of water, and causing flooding[57].
The results from the analysis show that the DEM data can be an accurate representation of drainage networks, which was derived from flow direction and slow accumulation for effective water resource management[60]. These findings were also corroborated by making use of DEM to produce several characteristic maps like slope, aspects and potential run-off coefficient for effective management of stormwater[61].
The final flood vulnerability map generated was found to be consistent with the historical flood events in the study area. The final flood vulnerability map is shown in Figure 5 below. Most of the highly flooded or flood prone areas were found in the low-lying parts of the metropolis especially the crowded flood plains which have been encroached upon by urban developments. The altitude of the study area ranges between 126 and 227 m above sea level and the low elevation which are located in the south-western and north central parts of the study area (altitude below 157 m above sea level are the most vulnerable areas to flood inundation and huge amount of stormwater).
The results also showed that elevated areas with steep slopes were relatively less susceptible to flood hazards[62,63]. Studies have shown that areas with flat and/or convex surface are relatively more susceptible to floods than convex or concave areas[64,65].
According to the NDVI represents the vegetation density over an area, and it is one of the factors used for determining flood vulnerability[66]. The NDVI maps were overlaid on the final flood map to evaluate the effects of the presence of vegetation (GI) and flood patterns in the study area. Researchers have shown that higher vegetation density decreases the velocity of the stormwater, urban runoff and flood inundation[4,5]. In this study (Figure 6) areas with NDVI values from - 0.37 to 0.06, 0.06 to 0.11, 0.11 to 0.14, 0.14 to 0.19, and 0.19 to 0.36 as very low, low, moderate, high, and very high, susceptible to flooding, respectively. Areas with high to very high NDVI values are found in the central and southeastern parts of the district. Similarly, a study conducted by Ali et al.[66] considered areas with NDVI values 0.51 as very high, high, moderate, low, and very low susceptibility to flooding respectively.
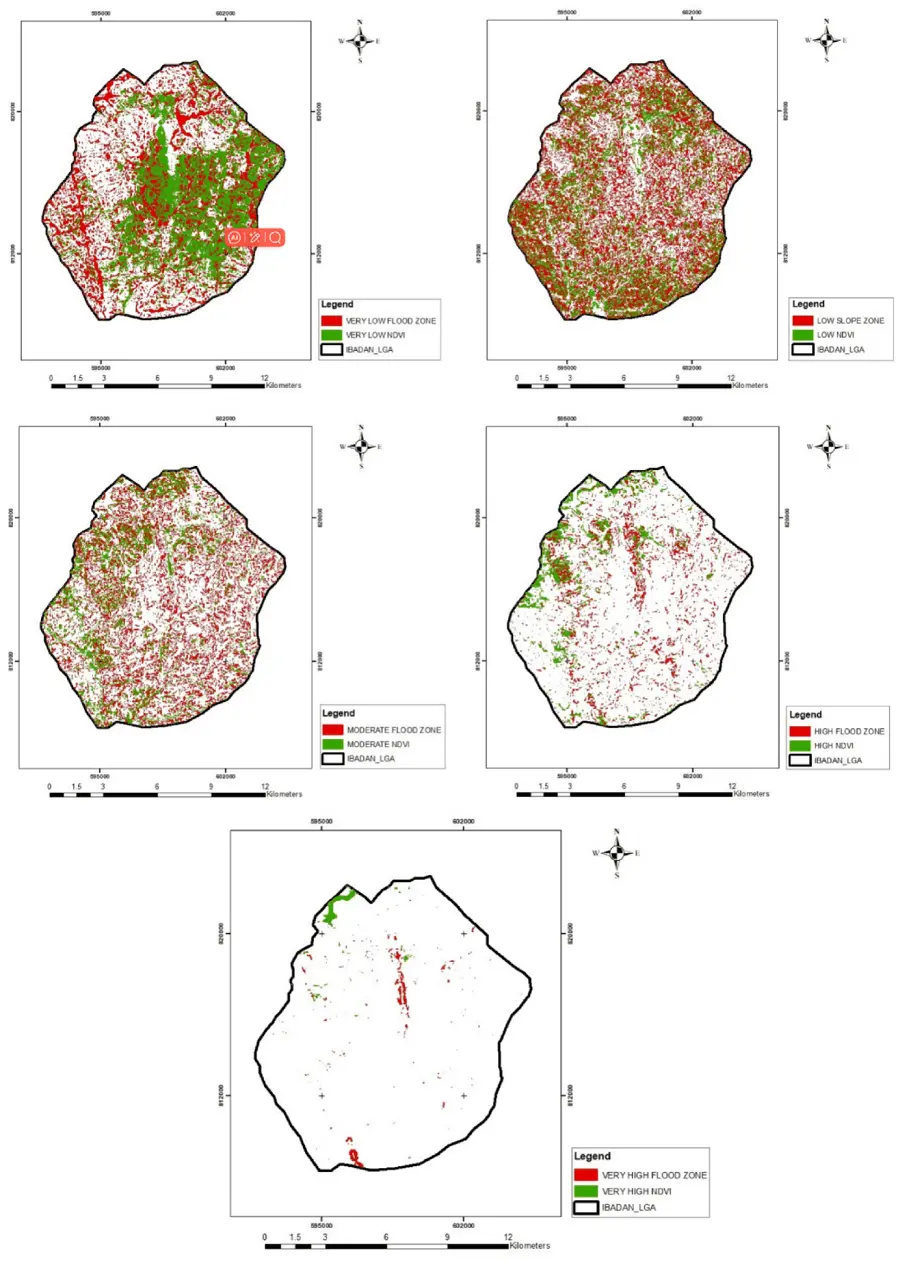
Figure 6. Overlay of flood zone and NDVI. NDVI: Normalized Difference Vegetation Index.
Green infrastructure can reduce the urban runoff and flooding, and creates resilience to manage environmental problems sustainably. Green infrastructure systems are more sustainable than conventional grey systems[67] in stormwater management most especially now when the financial resources are not available for construction of pipes, gutters, ditches, storm sewers etc. stressed further that green space inside the flood plain is more effective than its existence outside the flood plain[68]. GI was found to be an effective service to mitigate flood impacts on urban environment.
4. Conclusion
The rapid population growth and urbanization witnessed in Ibadan metropolis have had profound effects on the general environmental conditions. The land use land cover analysis revealed that the green infrastructure is decreasing at alarming rates with haphazard developments springing up at any available spaces within the city and surrounding areas. The green infrastructure in the city is being degraded and modified which increases the runoff and flooding. Urban development in Ibadan has drastically changed the green infrastructure to impervious surfaces. Majority of those areas with low NDVI corresponded with those areas with high vulnerability to flooding and high amount of stormwater. This shows the importance green infrastructures as valuable tool that can be used to manage rainfall discharge due to urban development and build resilience against stormwater in various parts of the city.
The study has shown that GI practices remain the most economical way to absorb and manage stormwaters because it reduces erosion along river banks; improve water quality through filtration; and increase groundwater recharge. It can be effective in reducing flooding and stormwater impacts in urban communities with the introduction of a watershed approach to planning instead of a plan that only accounts for a city's portion of the flooding or a subwatershed. With the growing frequency and severity of climate-related natural disasters, it is crucial to have access to dynamic and accurate stormwater mapping. Stormwater mapping is usually conducted by cities to better understand how climate events will affect their community infrastructure and thus help them to make informed planning decisions based on their findings. Stormwater mapping requires comprehensive, accurate, timely and up-to-date data. With the advent of geospatial techniques, it is increasingly possible for urban planners to help cities develop climate resilience strategies in a rapidly changing world.
5. Recommendations
Based on the results of the study it is recommended that the city administrators and planners should adopt of a holistic approach that integrates multiple stormwater management and mitigation strategies, including, resilient stormwater GI that will minimize risks in the study area. Relevant data can be sourced to map and derive actionable insights from high-resolution, up-to-date geospatial imagery, land cover data, impervious and pervious surfaces, and the first-party stormwater data of the city. GI which was overlaid with other land use maps revealed that its effective use can help to provide adequate solution to these stormwater problems. These data could be used in urban planning as a starting point to develop resilience-based GI infrastructure development plans and stormwater mitigation designs at an urban scale. Since GI is less disruptive to the hydrologic cycle, efforts should be made by the government and non-government organizations to make local residents to be aware and encouraged to maintain and plant greens in order to reduce impermeable surfaces. Future research should use high resolution satellite imagery and other cutting-edge analytical techniques to enhance the effectiveness, accuracy, and reliability of the model outputs. Given the limitations of this study it is important to develop more accurate estimation models and finer resolution of NDVI datasets should be considered for the study area because they influence the values of important spatial indices.
Authors Contributions
Busari RS , Adedeji OH: Data curation, formal analysis, writing-original draft, writing-review & editing. All authors approved the final version of the manuscript.
Conflicts of Interest
The authors declare no conflicts of interest.
Ethical approval
Not applicable.
Consent to participate
Not applicable.
Consent to publication
Not applicable.
Availability of data and material
Not applicable.
Funding
None.
Copyright
© The Author(s) 2024.
References
-
1. United Nations Department of Economic and Social Affairs. World urbanization prospects: the 2018 revision. New York: United Nations; 2018 [cited 2024 Apr 19].
-
2. Kim HW, Li MH. Sustainable stormwater management: examining the role of local planning capacity in mitigating peak surface runoff. Sustainability. 2016;8(9):763.
[DOI] -
3. United States Environmental Protection Agency (USEPA) [Internet]. Technical guidance on implementing the stormwater runoff requirements for federal projects under Section 438 of EISA; 2009 [cited 2024 May 5]. Available from: https://www.epa.gov/sites/default/files/2015-08/documents/epa_swm_guidance.pdf
-
4. Bell CD, Spahr K, Grubert E, Stokes-Draut J, Gallo E, McCray JE, et al. Decision making on the gray-green stormwater infrastructure continuum. J Sustainable Water Built Environ. 2019;5(1):04018016.
[DOI] -
5. Conley G, McDonald RI, Nodine T, Chapman T, Holland C, Hawkins C, et al. Assessing the influence of urban greenness and green stormwater infrastructure on hydrology from satellite remote sensing. Sci Total Environ. 2022;817:152723.
[DOI] -
6. Patra S, Sahoo S, Mishra P, Mahapatra SC. Impacts of urbanization on land use/cover changes and its probable implications on local climate and groundwater level. J Urban Manage. 2018;7(2):70-84.
[DOI] -
7. Mshelia SS. Assessment of wastewater management in Kano metropolis, Kano State, Nigeria. Int J Res Trends Soc Sci. 2024,2(1):1-18. Available from: https://www.researchgate.net/publication/378033690
-
8. Cities WS. Blueprint 2011 stormwater management in a water sensitive city. The Centre for Water Sensitive Cities. Clayton: Monash University; 2011. Available from: https://research.monash.edu/en/publications/2013
-
9. Andersson E. Urban landscapes and sustainable cities. Ecol Soc. 2006;11(1):34.
[DOI] -
10. Lundholm JT, Richardson PJ. MINI-REVIEW: Habitat analogues for reconciliation ecology in urban and industrial environments. J Appl Ecol. 2010;47(5):966-975.
[DOI] -
11. Carlyle-Moses DE, Livesley S, Baptista MD, Thom J, Szota C. Urban trees as green infrastructure for stormwater mitigation and use. In: Levia DF, Carlyle-Moses DE, Iida S, Michalzik B, Nanko K, Tischer A, editors. Forest-Water Interactions. Cham: Springer; 2020. p. 397-432.
[DOI] -
12. Das PC, Esraz-Ul-Zannat M. Assessing the impacts of land use-land cover changes on direct surface runoff: A remote sensing approach in Khulna City. Water Sci Technol. 2022;85(10):3122-3144.
[DOI] -
13. Copeland C. Green infrastructure issues in managing urban stormwater. Congressional Research Service; 2016. Available from: http://www.nationalaglawcenter.org/wp-content/uploads/assets/crs/R43131.pdf
-
14. McPhillips LE, Matsler M, Rosenzweig BR, Kim Y. What is the role of green stormwater infrastructure in managing extreme precipitation events? Sustain Resil Infrastruct. 2021;6(3-4):133-142.
[DOI] -
15. Hoover FA, Meerow S, Grabowski ZJ, McPhearson T. Environmental justice implications of siting criteria in urban green infrastructure planning. J Environ Policy Plann. 2021,23(5):665-682;
[DOI] -
16. Uribe CHA, Brenes RB, Hack J. Potential of retrofitted urban green infrastructure to reduce runoff-A model implementation with site-specific constraints at neighborhood scale. Urban For Urban Gree. 2022;69:127499.
[DOI] -
17. Wang Y, Meng F, Liu H, Zhang C, Fu G. Assessing catchment scale flood resilience of urban areas using a grid cell based metric. Water Res. 2019;163:114852.
[DOI] -
18. Liu Z, Xiu C, Ye C. Improving urban resilience through green infrastructure: an integrated approach for connectivity conservation in the Central City of Shenyang, China. Complexity. 2020;2020(1):1-15.
[DOI] -
19. Mosleh L, Negahban-Azar M, Pavao-Zuckerman M. Stormwater Green Infrastructure Resilience Assessment: A Social-Ecological Framework for Urban Stormwater Management. Water. 2023;15(9):1786.
[DOI] -
20. Jefferson AJ, Bhaskar AS, Hopkins KG, Fanelli R, Avellaneda PM, McMillan SK. Stormwater management network effectiveness and implications for urban watershed function: A critical review. Hydrol Process. 2017;31(23):4056-4080.
[DOI] -
21. Qumba B, Nkhonjera GK, Alowo R. An investigation of past and present stormwater management practices within the City of Johannesburg. In: TIOP Conference Series: Earth and Environmental Science. The 5th International Workshop on Environment and Geoscience; 2022 Jul 16-18; Online. Bristol: IOP Publishing. 2022;1087(1):012024.
[DOI] -
22. Fletcher TD, Shuster W, Hunt WF, Ashley R, Butler D, Arthur S, et al. SUDS, LID, BMPs, WSUD and more-The evolution and application of terminology surrounding urban drainage. Urban Water J. 2015;12(7):525-542.
[DOI] -
23. Berland A, Shiflett SA, Shuster WD. Garmestani AS, Goddard HC, Herrmann DL, et al. The role of trees in urban stormwater management. Landsc Urban Plan. 2017;162:167-177.
[DOI] -
24. Rodriguez M, Fu G, Butler D, Yuan Z, Sharma K. Exploring the spatial impact of green infrastructure on urban drainage resilience. Water. 2021;13(13):1789.
[DOI] -
25. Zischg J, Zeisl P, Winkler D, Rauch W, Sitzenfrei R. On the sensitivity of geospatial low impact development locations to the centralized sewer network. Water Sci Technol. 2018,77(7-8):1851-1860;
[DOI] -
26. Viti M, Löwe R, Sørup HJD, Rasmussen M, Arnbjerg-Nielsen K, McKnight US. Knowledge gaps and future research needs for assessing the non-market benefits of Nature-Based Solutions and Nature-Based Solution-like strategies. Sci Total Environ. 2022;841:156636.
[DOI] -
27. Urban Land Institute [Internet]. Ten Principles for Building Resilience about the Urban Land Institute; Washington: Urban Land Institute; 2018. Available from: https://americas.uli.org/ten-principles-building-resilience/
-
28. Giese E, Shirmohammadi A, Rockler A, Pavao-Zuckerman MA. Assessing Watershed-Scale Stormwater Green Infrastructure Response to Climate Change in Clarksburg, Maryland. J Water Resour Plan Manag. 2019;145(10):05019015.
[DOI] -
29. Raheem WM, Adeboyejo AT. Urban greening and city sustainability in Ibadan metropolis, Nigeria. Ethiop J Environ Stud Manage. 2016;9(3):287-302.
[DOI] -
30. Areola AA, Ikporukpo CO. Social ecology and urban green spaces in Ibadan, Nigeria. J Appl Sci Environ Manage. 2018;22(7):1111-1120.
[DOI] -
31. Meerow S, Newell JP. Spatial planning for multifunctional green infrastructure: Growing resilience in Detroit. Landsc Urban Plan. 2017;159:62-75.
[DOI] -
32. Samora-Arvela A, Ferrão J, Ferreira J, Panagopoulos T, Vaz E. Green infrastructure, climate change and spatial planning: learning lessons across borders. J Spatial Organ Dyn. 2017;5(3):176-188. Available from: https://run.unl.pt/bitstream/10362/31098/1/selection_8_.pdf
-
33. Staddon C, Ward S, De Vito L, Zuniga-Teran A, Gerlak AK, Schoeman Y, et al. Contributions of green infrastructure to enhancing urban resilience. Environ Syst Decisions. 2018;38:330-338.
[DOI] -
34. Pamukcu-Albers P, Ugolini F, La Rosa D, Gradinaru SR, Azevedo JC, Wu J. Building green infrastructure to enhance urban resilience to climate change and pandemics. Landscape Ecol. 2021;36(3):665-673.
[DOI] -
35. Zakka SD, Permana AS, Majid MR, Danladi A, Bako PE. Urban greenery a pathway to environmental sustainability in Sub Saharan Africa: a case of northern Nigeria cities. Int J Built Environ Sustainability. 2017;4(3):180-189.
[DOI] -
36. Wahab B. Ibadan: A rapidly growing city in need of a Master Plan.
-
37. Jimoh R, Aronja Y, Albert C, Amoo N. Spatio-temporal urban expansion analysis in a growing city of Oyo town, Oyo State, Nigeria using remote sensing and geographic information system (GIS) tools. Int J of Environ Geoinformatics. 2018;5(2):104-113.
[DOI] -
38. Umukiza E, Raude JM, Wandera SM, Petroselli A, Gathenya JM. Impacts of land use and land cover changes on peakdischarge and flow volume in Kakia and Esamburmbur sub-catchments of Narok Town, Kenya. Hydrology. 2021;8(2):82.
[DOI] -
39. Agbola T. Master Plan for Ibadan. Speech delivered at 2013 Adegoke Adelabu Memorial Lecture, 2013.
-
40. Makinde OO. Housing delivery system, need and demand. Environ Dev Sustain. 2014;16:49-69.
[DOI] -
41. Popoola AS. Spatial Variations in Housing Condition and Residents' Health in Area Prone to Flood in Ibadan Metropolis, Nigeria [dissertation]. Nigeria: Obafemi Awolowo University; 2013.
-
42. Adedeji OH, Odufuwa BO, Adebayo OH. Building capabilities for flood disaster and hazard preparedness and risk reduction in Nigeria: need for spatial planning and land management. J Sustainable Dev Africa. 2012;14(1):45-58. Available from: https://jsd-africa.com/Jsda/Vol14No1-Spring2012A/PDF
-
43. Olawuni OP, Popoola AS, Bolukale AT, Eluyele KP, Adegoke JO. An assessment of the factors responsible for flooding in Ibadan metropolis, Nigeria. J Environ Earth Sci. 2015;5:1-7. Available form: https://www.iiste.org/Journals/index.php/JEES/article/view/27039/27722
-
44. Saraswat C, Kumar P, Mishra BK. Assessment of stormwater runoff management practices and governance under climate change and urbanization: An analysis of Bangkok, Hanoi and Tokyo. Environm Sci Policy. 2016;64:101-117.
[DOI] -
45. Dimitrov S, Georgiev G, Georgieva M, Gluschkova M, Chepisheva V, Mirchev P, et al. Integrated assessment of urban green infrastructure condition in Karlovo urban area by in-situ observations and remote sensing. One Ecosyst. 2018;3:e21610.
[DOI] -
46. Weng Q. Modeling urban growth effects on surface runoff with the integration of remote sensing and GIS. Environ Manage. 2001;28:737-748.
[DOI] -
47. Population Hub, 2024. Population of Ibadan. 2024. Available from: https://www.worldometers.info/nigeria-population/
-
48. LIONS@FRICA [Internet]. Available from: https://www.lionsafrica.org/
-
49. Jensen JR. Introductory digital image processing: A remote sensing perspective. 3rd ed. London: Pearson; 2005.
-
50. Loranty MM, Davydov SP, Kropp H, Alexander HD, Mack MC, et al. Vegetation indices do not capture forest cover variation in upland Siberian larch forests. Remote Sensing. 2018;10(11):1686.
[DOI] -
51. Huang S, Tang L, Hupy JP, Wang Y, Shao G. A commentary review on the use of normalized difference vegetation index (NDVI) in the era of popular remote sensing. J For Res. 2021;32(1):1-6.
[DOI] -
52. Kim HW, Kim JH, Li W, Yang P, Cao Y. Exploring the impact of green space health on runoff reduction using NDVI. Urban For Urban Green. 2017;28:81-87.
[DOI] -
53. Riazi M, Khosravi K, Shahedi K, Ahmad S, Jun C, Bateni SM, et al. Enhancing flood susceptibility modeling using multi-temporal SAR images, CHIRPS data, and hybrid machine learning algorithms. Sci Total Environ. 2023;871:162066.
[DOI] -
54. Li Z, Tang X, Li L, Chu Y, Wang X, Yang D. GIS-based risk assessment of flood disaster in the Lijiang River Basin. Sci Rep. 2023,13(1):6160;
[DOI] -
55. Liang Y, Song W. Integrating potential ecosystem services losses into ecological risk assessment of land use changes: A case study on the Qinghai-Tibet Plateau. J Environ manag. 2022;318:115607.
[DOI] -
56. Paule-Mercado MCA, Salim I, Lee BY, Memon S, Sajjad RU, Sukhbaatar C, et al. Monitoring and quantification of stormwater runoff from mixed land use and land cover catchment in response to land development. Ecol Indic. 2018;93:1112-1125.
[DOI] -
57. Dash P, Sar J. Identification and validation of potential flood hazard area using GIS-based multi-criteria analysis and satellite data-derived water index. J Flood Risk Manag. 2020;13(3):e12620.
[DOI] -
58. Ajibade FO, Ajibade TF, Idowu TE, Nwogwu NA, Adelodun B, Lasisi KH, et al. Flood-prone area mapping using GIS-based analytical hierarchy frameworks for Ibadan city, Nigeria. J Multi-Criteria Decis Anal. 2021;28(5-6):283-295.
[DOI] -
59. Negese A, Worku D, Shitaye A, Getnet H. Potential flood-prone area identification and mapping using GIS-based multi-criteria decision-making and analytical hierarchy process in Dega Damot district, northwestern Ethiopia. Appl Water Sci. 2022;12(12):255.
[DOI] -
60. Sibomana A, Bhat HG. Drainage network extraction from ASTER G DEM 1 arc-second resolution using D8 model for Kagunuzi watershed in Kibira national park north-western of Burundi. Interl J Curr Res Life Sci. 2018;7(04):1897-1902. Available from: https://www.academia.edu/99915973
-
61. Mahmoud SH, Alazba AA, Adamowski J, El-Gindy AM. GIS methods for sustainable stormwater harvesting and storage using remote sensing for land cover data-location assessment. Environ Monit Assess. 2015;187(9):598.
[DOI] -
62. Zzaman RU, Nowreen S, Billah M, Islam AS. Flood hazard mapping of Sangu River basin in Bangladesh using multi-criteria analysis of hydro-geomorphological factors. J Flood Risk Manage. 2021;14(3):e12715.
[DOI] -
63. Majeed M, Lu L, Anwar MM, Tariq A, Qin S, El-Hefnawy ME, et al. Prediction of flash flood susceptibility using integrating analytic hierarchy process (AHP) and frequency ratio (FR) algorithms. Front Environ Sci. 2022;10:1037547.
[DOI] -
64. Ullah K, Zhang J. GIS-based flood hazard mapping using relative frequency ratio method: A case study of Panjkora River Basin, eastern Hindu Kush, Pakistan. Plos one. 2020;15(3):e0229153.
[DOI] -
65. Amen AR, Mustafa A, Kareem DA, Hameed HM, Mirza AA, Szydlowski M, et al. Mapping of flood-prone areas utilizing GIS techniques and remote sensing: a case study of Duhok, Kurdistan Region of Iraq. Remote Sens. 2023;15(4):1102.
[DOI] -
66. Ali SA, Parvin F, Pham QB, Vojtek M, Vojteková J, Costache R, et al. GIS-based comparative assessment of flood susceptibility mapping using hybrid multi-criteria decision-making approach, naïve Bayes tree, bivariate statistics and logistic regression: a case of Topľa basin, Slovakia. Ecol Indic. 2020;117:106620.
[DOI] -
67. Chowdary NY. Sustainable stormwater management with utilization of green infrastructure [dissertation]. Hydrabad: Jhafa University; 2019.
-
68. Kim HY. Analyzing green space as a flooding mitigation-storm Chaba case in South Korea. Geomatics Nat Hazards Risk. 2021;12(1):1181-1194.
[DOI]
Copyright
© The Author(s) 2024. This is an Open Access article licensed under a Creative Commons Attribution 4.0 International License (https://creativecommons.org/licenses/by/4.0/), which permits unrestricted use, sharing, adaptation, distribution and reproduction in any medium or format, for any purpose, even commercially, as long as you give appropriate credit to the original author(s) and the source, provide a link to the Creative Commons license, and indicate if changes were made.
Share And Cite