Abstract
This study investigates the integration of Building Information Modeling (BIM) and the Internet of Things (IoT) in construction projects to enhance efficiency, safety, and lifecycle monitoring. Despite the significant potential of BIM and IoT to facilitate the transformation of the construction industry, existing research lacks a comprehensive framework that addresses its interoperability challenges, data security concerns, and real-world implementation barriers. To address this gap, a multiple case study approach was employed, incorporating semi-structured interviews, document analysis, and real-world project evaluations. The results find that standardized protocols, data encryption, and modular IoT devices are essential for effective BIM-IoT adoption across different construction phases. The proposed framework offers a structured approach for construction teams to effectively utilize BIM and IoT, facilitating smarter and more sustainable project management. The contribution of this paper resides in the detailed analysis of the BIM-IoT applications, clearly demonstrating its advantages, such as improving building productivity and safety, and its potential to align with China's sustainable urban development goals. The study also provides a forward-looking prediction of the future development trend of BIM-IoT in China, offering valuable insights for construction project teams and decision makers. By demonstrating the structured framework and its practical application, this study provides guidance for the industry's transition to smarter and safer construction practices.
Keywords
1. Introduction
The construction industry is undergoing a technological revolution. Building Information Modeling (BIM) and Internet of Things (IoT) are two key drivers for change[1]. Building Information Modeling, founded on the creation of comprehensive 3D virtual models has transformed the establishment, visualization and management of construction projects[2]. The IoTs, realized by interconnected device networks, has ushered in a new era of on-the-ground information collection and analysis[1]. It ensures the building's operation is both efficient and sustainable. BIM-IoT is developing rapidly in China. Driven by government policies, BIM-IoT has been applied to both general construction projects and large-scale infrastructure construction.
Existing research has recognized the potential of BIM-IoT integration for improving efficiency, safety, and data-driven decision-making in construction. However, most studies focus on isolated aspects rather than a comprehensive lifecycle approach that spans all project phases. Furthermore, challenges related to interoperability, data security, and the lack of standardized frameworks hinder large-scale adoption. This study addresses these gaps by systematically analyzing multiple real-world case studies to develop a holistic BIM-IoT application framework. The proposed framework is designed as a strategic guide for construction companies to harness BIM-IoT effectively, drive technological innovation, and prepare for the future of digital construction.
The findings demonstrate the powerful impact of BIM-IoT on productivity, quality management, and resource optimization, highlighting both current practices and emerging trends within China's rapidly evolving construction landscape. The proposed framework provides practical strategies for overcoming integration challenges, ensuring secure data exchange, and optimizing construction processes. By offering a structured approach, this research contributes to the advancement of digital construction management, equipping industry stakeholders with a roadmap for implementing BIM-IoT in diverse project environments.
2. Literature Review
In the lifecycle of a construction project, three main stages can usually be identified: design, construction, and operation[3]. During the design phase, BIM-IoT facilitates real-time collaboration and optimizes processes, while in construction, it enhances material management, safety, and maintenance activities through IoT data collection and BIM monitoring. The operation stage benefits from real-time monitoring for efficiency and energy savings, with BIM-IoT providing early warnings for disaster management.
2.1 Application of BIM-IoT in design stage
Designers frequently collaborate digitally using platforms like ArchiCAD, Revit and SketchUp to create and modify virtual models[3], relying solely on BIM presents the challenge of not being able to identify problems in a timely manner. Numerous issues remained undiscovered until construction commenced[4]. BIM-IoT has great potential for real-time communication and collaboration[5]. It facilitates timely interaction that addresses the limitations of BIM in the design process and optimizes workflows across the industry[1].
During the project design phase, architects collaborate to develop BIM models,, which provide the client with final design and pricing information, facilitating contractor selection and project initiation[3]. The integration of IoT and BIM can capture more accurate construction site data, leading to a more precise BIM model[6]. BIM-IoT can also incorporate weather conditions and natural light availability data on the construction site before construction begins, helping improve energy efficiency and promote environmental sustainability[7].
In the component production process, BIM-IoT records the entire production process and collects real-time data, facilitating coordination between upstream design and downstream construction processes[8]. Also, sensors can be incorporated into the components to facilitate accurate identification of their installation location[9] and reduce the risk of delivery errors to other construction sites[10]. Additionally, BIM-IoT can accurately calculate the transportation time, helping field managers to optimize schedule and save more time[11].
2.2 Application of BIM-IoT in construction stage
During the project construction phase, IoT systems facilitate the collection of diverse data, while the BIM systems enable effective implementation monitoring and data acquisition for construction projects[12,13]. This integration significantly contributes to material management and on-site maintenance activities. Furthermore, BIM-IoT integration enhances security supervision efficiency[12,14,15], schedule management effectiveness[6], and communication between personnel[1].
Sensors installed inside Personal Protective Equipment (PPE) can measure the extent of workers' compliance with the PPE rule[6] and continuously monitor workers' postures, physical condition and levels of fatigue, enabling the detection of falls from dangerous heights and ensuring their safety at all times[6,12,13,16,17]. With real-time monitoring of machine position and BIM simulation, the sensors installed on machinery on construction sites are able to identify safe areas and provide valuable insights into machine density and the detection of environmental conditions[18].
The on-site sensors, such as cameras, could be used to increase efficiency and safety and reduce monitoring costs[14]. Sensors installed on the construction site can serve as alarm systems for hazardous areas, effectively preventing personnel from approaching dangerous zones and reducing accidents[12,15]. Furthermore, on-site sensors can also monitor natural conditions such as humidity and temperature, thereby facilitating efficient site management[19].
2.3 Application of BIM-IoT in operation stage
During the project operational phase, BIM-IoT enables real-time monitoring of its operational status, leading to improved efficiency[20,21] and energy savings[22]. It can provide early warnings and optimal rescue plans in the event of disasters such as fires and earthquakes. In addition, BIM-IoT can monitor the extent of building damage, mitigate safety issues caused by long-term use, and prompt managers to carry out maintenance in a timely manner or take appropriate preventive measures[23].
2.4 Research gap derived from the previous research
While previous studies have demonstrated the potential benefits of BIM-IoT integration, several critical gaps remain unaddressed. Firstly, most of the existing research focuses on isolated aspects of BIM-IoT, such as safety monitoring or resource management, without presenting a unified framework that encompasses all phases of a construction project. This fragmented approach limits the practical application of BIM-IoT technologies, as real-world implementation requires a comprehensive and lifecycle-based approach.
Secondly, interoperability remains a persistent challenge. Many studies highlight the advantages of integrating BIM and IoT but fail to address the technical and organizational barriers that hinder seamless data exchange across different platforms and stakeholders. Without standardized protocols and clear guidelines, large-scale implementation remains inconsistent and inefficient.
Thirdly, data security concerns are often overlooked. As BIM-IoT systems collect and transmit vast amounts of sensitive project data, the risk of cyber threats, data breaches, and unauthorized access increases. While some research highlights encryption techniques and secure data-sharing mechanisms, the lack of consensus on best practices hampers the widespread adoption of secure BIM-IoT frameworks.
Furthermore, while BIM-IoT adoption in China has been growing due to supportive government policies, few studies explore how these insights can be adapted to different regulatory and market environments. This limitation restricts the global applicability of existing research, as construction practices, standards, and legal requirements vary across regions.
To address these gaps, this study develops a holistic BIM-IoT application framework based on a multi-case study approach. By systematically analyzing real-world projects, the research provides a structured approach for the effective adoption of BIM-IoT, addressing integration challenges, data security concerns, and interoperability barriers. This framework serves as a practical guide for industry professionals and policymakers, ensuring the effective implementation of BIM-IoT across diverse construction environments.
3. Methodology
The research was carried out in two main phases, namely the literature review and multi-case study analysis. The literature review aimed to explore general applications of BIM-IoT in the design, construction and operation phases of construction projects. The multi-case study approach was adopted to investigate the specific applications of BIM-IoT for real construction projects in China. The case study method serves as an empirical investigation technique, enabling an in-depth examination of BIM-IoT within specific projects and facilitating the rich analysis of research data from various perspectives[24].
Purposive data collection was utilized in this study, to intentionally select cases that provide rich and relevant information[25]. The selected case studies meet key criteria to ensure their representativeness in examining BIM-IoT integration across diverse construction environments. Each project involves BIM-IoT implementation at multiple stages, features distinct technical challenges, and represents a different sector: infrastructure (underground utility tunnel), commercial (winter resort), and industrial (LNG terminal). These cases illustrate the adaptability of BIM-IoT solutions in varying contexts, making them suitable benchmarks for evaluating industry-wide trends and best practices. A purposive sampling approach was used to select 7 industry professionals, including BIM specialists and IoT engineers, all of whom had direct experience with BIM-IoT integration in construction in China, and have participated in at least three BIM-related projects. The profiles of participants is included in Table 1.
Participants | Job Title | Relevant Working Time | Job Content | Affiliated unit |
1 | Supervising Engineer | 3 years | Responsible for BIM related matters | Construction Team |
2 | Head of technical center | 3 years | Responsible for the digital work of BIM, including data collection, analysis, etc. | Sensor manufacturer |
3 | Technical center supervisor engineer | 4 years | Ensure the correct implementation of BIM technology, coordinate with other departments, conduct software training and maintain BIM system | Construction Team |
4 | Supervising Engineer | 7 years | BIM production in the bidding stage, BIM related work in the construction process | Construction Team |
5 | Senior engineer | 10 years | Manages intricate projects, mentors junior staff, and upholds engineering standards. | Construction Team |
6 | Supervising Engineer | 8 years | Ensures technical standards are met, and oversees the design and implementation phases | Construction Team |
7 | Senior engineer | 13 years | Engages in port engineering design, BIM consulting work | Design Team |
BIM: Building Information Modeling.
To collect data for a specific BIM-IoT project, in-depth semi-structured interviews were conducted, a predetermined set of open-ended questions were designed to prompt discussion with the flexibility for the interviewer to delve deeper into specific themes or responses[26]. For this study, the interviewees were presented with a standardized set of 10 questions, as presented in Appendix, concerning specific construction projects. The interview questions were refined through a pilot test with two experts. Each interview lasted approximately 45-60 minutes and followed a semi-structured format, allowing for flexibility while maintaining a focus on key themes such as implementation challenges, benefits, and future trends. The interviewees provided not only rich interview data but also supplementary documents related to the projects.
Following each interview, the interviewer systematically analyzed the interview data along with the document analysis, those data were reviewed and categorized based on key themes such as challenges, benefits, and implementation strategies. Common patterns across different case studies were identified to extract meaningful insights. The findings were then compared with existing literature to validate their relevance. This approach ensured that the conclusions were well-supported and applicable to real-world construction scenarios.
A within-case analysis was conducted to independently evaluate the BIM-IoT application in each project. Then, a cross-case analysis was performed, which involved a comprehensive exploration of similarities and differences across cases, aiming to support empirical generalizability and theoretical predictions[27]. The cross-case analysis serves as an analytical approach to leverage knowledge derived from the individual case studies. Ultimately, the findings from these cases are consolidated to develop a BIM-IoT application framework, which was sent to the interviewees for validation, including BIM experts, IoT engineers, and construction managers involved in the studied projects. Their feedback highlighted the practical feasibility of the framework while suggesting refinements in data security measures, sensor standardization, and integration protocols. These insights were incorporated into the final framework by enhancing security strategies, refining interoperability guidelines, and adjusting implementation workflows to better align with real-world constraints. This iterative validation process strengthened the framework's relevance and usability, ensuring its adaptability to diverse construction environments.
4. Case Study Analysis
4.1 Case 1
4.1.1 Project introduction
The underground utility tunnel, crucial for underground traffic, is vast and intricate, posing numerous challenges for the project team. Situated in a climate with seasonal rainfall, the project faces issues like drainage and structural integrity and tight deadlines. The workload is substantial, with a complex underground setup demanding precise management and risk mitigation measures. Given the substantial workload, accurate component management and real-time data are essential for ensuring safety and quality. To resolve these challenges, the project team has adopted BIM-IoT to enhance efficiency and quality.
4.1.2 Sensors used in the project
BIM technology implements integrated and visual management of utility tunnels, providing accurate data of tunnel components and construction information. IoT technology enables real-time data collection and monitoring on the construction site, including parameters such as temperature, humidity and pressure.
Temperature sensors: Temperature sensors installed in key locations can monitor temperature changes in real time. These temperature data is transmitted to the central management system, allowing construction personnel to adjust measures according to the data to ensure temperature control and quality management.
Humidity sensors: Humidity sensors on site can monitor changes in humidity on-site in real time and transmit the data to a central management system for analysis. These data help construction personnel to promptly assess humidity fluctuations and make necessary construction adjustments and protective measures.
Pressure sensors: Pressure sensors installed in key locations can sense pressure changes on the tunnel structure in real time. This data is transmitted to a central management system, enabling construction personnel to promptly detect anomalies and take appropriate measures to ensure construction quality and structural safety.
Water level sensors: Water level sensors installed in wells or drains can detect changes in the water table in real time. The data is transmitted to a central management system, allowing construction personnel to design drainage solutions and make construction adjustments based on water level information, ensuring the smooth progress of underground construction.
Radio-frequency identification (RFID): In utility tunnel construction, BIM generates 3D models for detailed component info and construction animations, aiding site management. RFID technology, with readers and chips, facilitates contactless data collection, guiding tunnel construction[28]. Integrating RFID with BIM allows real-time management and planning of utility tunnel layouts and construction processes.
4.1.3 Application process of BIM-IoT
According to the interviewee's description, the application process of BIM-IoT in the project can be summarized in Figure 1.

Figure 1. Application process of BIM-IoT of Case 1. BIM: Building Information Modeling; IoT: Internet of Things.
Objective Definition: The team sets clear project goals to boost efficiency, resource use, and safety, and pinpoints vital parameters like temperature, humidity, pressure, and water levels for monitoring.
Network Configuration: The team selects the appropriate wireless sensor network technology, considering communication modes and transmission protocols between sensors. Relay devices of the sensor network and the path of data transmission should be determined to ensure the stable transmission of signals.
Sensor Selection: BIM team selects appropriate sensor devices based on project requirements and design, including temperature, humidity, pressure, and water level sensors. They define installation positions and methods for each sensor device, and ensures that the sensors can accurately sense the required parameters and interact with the central management system.
Centralized Management: BIM engineers develop a central system for sensor data collection, storage, and analysis, ensuring data security through database structuring and communication setup. They implement standardized formats for data sharing and establish alarms for data exceeding set limits.
Data Security: The BIM team implements data security and privacy protection measures, including data encryption, access control, backup, and disaster recovery, and conducts compliance audits and monitoring of sensor data to ensure data integrity and traceability.
Maintenance Inspection: The team regularly checks the operating status and performance of the sensor equipment.
4.1.4 Challenges of Using BIM-IoT in the project
Although the benefits of using BIM-IoT are evident, its application in this project also presented several challenges. Firstly, the project faced the challenge of integrating BIM and IoT technologies, which lacked a unified standard, leading to potential compatibility issues between different systems and hindering seamless data communication. This affected the efficiency of collaborative efforts among various stakeholders. Secondly, there was the challenge of data security and privacy during the collection and sharing process, where the risk of data leakage or misuse posed a significant threat to the project's integrity and the safety of its participants. If the sensor's data is compromised, it could result in incorrect assessments of safety conditions and lead to potential construction hazards or accidents.
4.1.5 Summary
The main results of the BIM-IoT applications in this project are summarized in Table 2, including its application areas, benefits, challenges, and solutions.
Project Type | BIM-IoT Applications | Benefits | Challenges | Solutions |
Underground Utility Tunnel | • Real-time monitoring with sensors • RFID for component tracking | • Enhanced safety • Improved project efficiency • Reduced material waste | • Interoperability issues • Data security risks • Complex underground setup | • Standardized protocols • Data encryption • Modular IoT devices • Rigorous maintenance checks |
RFID: radio-frequency identification; BIM: Building Information Modeling; IoT: Internet of Things.
4.2 Case 2
4.2.1 Project introduction
The winter resort, 100 km from downtown, spans 58,100 sqm with five north-facing clusters, using 9,400 tons of steel over 733 days. The project team faces two main challenges: a varied terrain with a 30-42m elevation and a complex perimeter structure demanding new construction methods. As China's first integrated land-use and contracting project, it pioneers BIM's lifecycle application, from design through operation. The site layout planning of the project is shown in Figure 2.
4.2.2 Sensors used in the project
The sensors used in the project played a significant role in ensuring construction safety, monitoring land changes, and enhancing management efficiency.
Inclinometers: Inclinometers measure ground or structural incline to evaluate soil deformation. Mounted on slopes, they detect changes in inclination, providing real-time stability monitoring and warnings.
Stress sensors: Stress sensors directly measure forces on soil or structures for real-time slope load monitoring, often installed at critical slope points to track changes in external forces.
Displacement sensors: Displacement sensors measure soil or structural movement, indicating soil deformation. They come in various types like laser, piezoelectric, and capacitive, each operating on distinct principles. In high-slope systems, these sensors are placed at key slope locations for real-time monitoring, offering deformation data and alerts.
The project utilizes IoT modules for real-time sensor data transmission to a smart platform, allowing for computer and mobile monitoring with alarm notifications. This boosts high-slope safety monitoring and ensures construction safety. Over 30 cameras feed into the platform for comprehensive site oversight, with AI detecting safety violations like improper PPE use, enhancing worker safety and construction process standardization.
The data from high-slope IoT monitoring, video security surveillance, labor management, quality safety, and other aspects can be imported into the project report template of the smart construction site system. This data collection method not only saves time for on-site management personnel in filling out reports but also reduces the likelihood of errors, enhancing the accuracy and reliability of the data.
4.2.3 Application process of BIM-IoT
According to the interviewee's description, the application process of BIM-IoT in the project can be summarized in Figure 3.

Figure 3. Application process of BIM-IoT of Case 2. BIM: Building Information Modeling; IoT: Internet of Things.
Precision Modeling: The team creates a BIM model incorporating the building and related elements like structures and pipelines, tailored to the project's terrain and climate, using 3D scanning for precision.
Strategic Sourcing: The team reaches out to sensor suppliers for detailed discussions on requirements and specs, sharing project details to ensure the suppliers grasp the needs and collaborate on finalizing the project's sensor requirements.
Contractual Agreement: Once the sensor supplier is determined, the team signs a formal contract with them, specifying the specifications, prices, delivery time, after-sales services, and other terms for the sensor devices, and clearly defines the warranty period, technical support, and training for the sensor devices in the contract.
Synchronized Deployment: The team coordinates a sensor installation timetable with construction activities for prompt deployment. Suppliers adhere to this timeline for delivery and setup, with technicians ensuring proper installation. Continuous communication with suppliers confirms that installations are up to standard.
Data Integration: Post-installation, the team works with suppliers to integrate sensors into the smart platform, ensuring precise data transmission, collection, storage, and analysis. They analyze data to spot issues and improvement chances, acting swiftly to refine processes.
Ongoing Optimization: The team sustains a partnership with the sensor supplier for ongoing performance reviews and BIM-IoT system enhancements. Regular sensor checks prevent malfunctions, and joint online monitoring ensures continuous data support for project phases.
4.2.4 Challenges of using BIM-IoT in the project
In the application of BIM-IoT within this project, several challenges have surfaced. Firstly, in slope monitoring systems, the deployment and maintenance costs of sensors can be high, especially in high-altitude mountainous environments where the installation and maintenance of sensors need to consider construction difficulties and costs. Additionally, the sheer volume of data produced by these sensors necessitates efficient transmission and processing to enable real-time monitoring, yet unstable network signals and slow transfer speeds can cause delays or data loss. The analysis and processing of sensor data require the establishment of effective algorithms and warning mechanisms to timely identify potential safety hazards on slopes and take appropriate measures. However, establishing accurate and reliable data analysis models may face challenges. Furthermore, difficulties in data exchange between 3D scanning technology and software like ArcGIS and Revit have been encountered, requiring a cumbersome process of drawing, annotating, and exporting data through CAD before it can be used with other applications, highlighting the need for more streamlined integration processes.
4.2.5 Summary
The main results of the BIM-IoT applications in project 2 are summarized in Table 3, including its application areas, benefits, challenges, and solutions.
Project Type | BIM-IoT Applications | Benefits | Challenges | Solutions |
Winter Resort | • High-slope safety monitoring using inclinometers, stress sensors, displacement sensors • AI-based worker safety compliance monitoring | • Improved safety and risk management, enhanced efficiency • Streamlined reporting | • High costs of deployment • Network instability • Complex terrain | • Cost-benefit analysis • Optimized sensor placement • Real-time data processing techniques |
BIM: Building Information Modeling; IoT: Internet of Things.
4.3 Case 3
4.3.1 Project introduction
The Port District-based project intends to build an essential Liquefied Natural Gas (LNG) terminal for the clean energy supply chain, including facilities like a ship berth and navigational aids. The construction faces challenges due to heavy components and harsh marine conditions, with a focus on safety and efficient labor management amidst a tight schedule. BIM-IoT integration with smart platforms overcomes these by synchronizing and managing various data streams, boosting construction quality and technical management.
4.3.2 Sensors used in the project
In the LNG terminal construction project, the application of BIM-IoT technology involves a variety of sensors that provide real-time data support for construction management.
Concrete temperature sensors: Concrete temperature sensors in the LNG terminal construction monitor real-time curing temps to avert quality defects from temperature extremes. The data is displayed on large screens via IoT platforms for easy monitoring and prompt responses.
Hydrodynamic wave sensors: Hydrodynamic wave sensors help the LNG terminal construction team gauge sea conditions for optimal work timing and design data. Stored and analyzed in a database, real-time results on a dashboard (Figure 4) aid in organizing construction and ensuring safety.
Environmental monitoring sensors: On-site sensors supply weather data crucial for safe construction, with real-time monitoring of temperature, wind speed, dust, and PM2.5. This data, integrated into a smart platform, enables statistical analysis to inform environmental management and improve decision-making.
RFID: RFID enhances safety by verifying worker's attendance and identities, ensuring access is restricted to authorized personnel. Linked with management systems and screens, it tracks employee movements and shifts, displaying analyzed data to support real-time project management and labor analysis.
Video surveillance cameras: On the LNG terminal job site, surveillance cameras capture the construction progress for real-time management oversight. IoT integrates this footage into a central dashboard, with geo-tagging for clear, online visibility of site activities.
4.3.3 Application process of BIM-IoT
In the LNG terminal construction project, the application steps of BIM-IoT technology can be summarized as the following key stages (Figure 5).

Figure 5. Application process of BIM-IoT of Case 3. BIM: Building Information Modeling; IoT: Internet of Things.
BIM in the Design Phase: The design team employed BIM to craft a 3D model for structural and weather resistance design, factoring in marine elements like waves, wind, and tides. Integration of oceanographic data simulates construction sea conditions, informing the plan scientifically.
Construction Simulation: BIM can simulate the working path of the construction ship, the material transport route, and the layout of temporary structures during construction. BIM models can be used for construction resource planning, including manpower, equipment and materials.
IoT Device Deployment and Data Analysis: Wave sensors, anemometers, video surveillance and RFID material tracking systems are installed to collect data about the construction environment and progress. The construction status and environmental changes can then be monitored in real time through data collected from IoT devices.
Construction and Quality Management: BIM engineers combine BIM models with IoT data for construction schedule and quality management. The BIM model can track the construction progress and compare with the planned progress; IoT data supports quality control of construction, such as monitoring the curing temperature of concrete.
Safety Risk Management: The management team uses BIM IoT systems for safety risk management of offshore construction. The weather changes and sea conditions can be monitored in real time, and warnings will be triggered when there are potential risks identified; The RFID and biometrics technologies are used to strengthen the safety management of field personnel.
Post-construction and Maintenance: The operations team can use BIM models for post-construction operation and maintenance planning of the terminal. BIM engineers create BIM models that contain construction and operational data to provide detailed asset information and maintenance guidance to maintenance teams.
4.3.4 Challenges of using BIM-IoT in the project
In this project, there are some difficulties in the application of BIM-IoT. First, offshore operations are greatly affected by weather, and natural factors such as wind, waves and salt spray pose challenges to the stability and life of construction equipment and sensors. The team selects sensors and equipment specifically designed for harsh offshore environments with anti-corrosion, water and dust resistance technologies. Second, offshore construction sites are often far from land, which complicates the logistics of material supply and personnel rotation. Third, the instability of offshore platforms increases construction safety risks, requiring real-time monitoring and rapid response mechanisms. Fourth, offshore construction must comply with strict environmental protection regulations to minimize the impact on the marine ecosystem. Fifth, communication signals in the offshore operation area may be weak or unstable, affecting the real-time transmission of data.
4.3.5 Summary
The main results of the BIM-IoT applications in project 3 are summarized in Table 4, including its application areas, benefits, challenges, and solutions.
Project Type | BIM-IoT Applications | Benefits | Challenges | Solutions |
LNG Terminal | • IoT for environmental monitoring • RFID for workforce management • BIM for construction simulation | • Better decision-making • Real-time hazard detection • Streamlined logistics | • Harsh marine conditions • Communication signal instability • Safety risks | • Corrosion-resistant sensors • Improved network infrastructure • Predictive maintenance planning |
IoT: Internet of Things; BIM: Building Information Modeling; LNG: Liquefied Natural Gas; RFID: radio-frequency identification.
5. Framework of BIM-IoT Application in Construction Projects
The three case studies discussed above all involve the application of BIM-IoT technology in large construction projects. These projects include various types, from underground utility tunnels, winter resorts, to liquefied natural gas terminals. Although the types of projects are different, there are common BIM-IoT application processes, benefits, challenges and corresponding strategies that can be generalized. Through cross-case analysis, this study proposes a theoretical framework of BIM-IoT application in construction projects, which covers the entire project lifecycle. The framework has been further validated and refined after incorporating experts' comments, and the finalized version is shown in Figure 6.
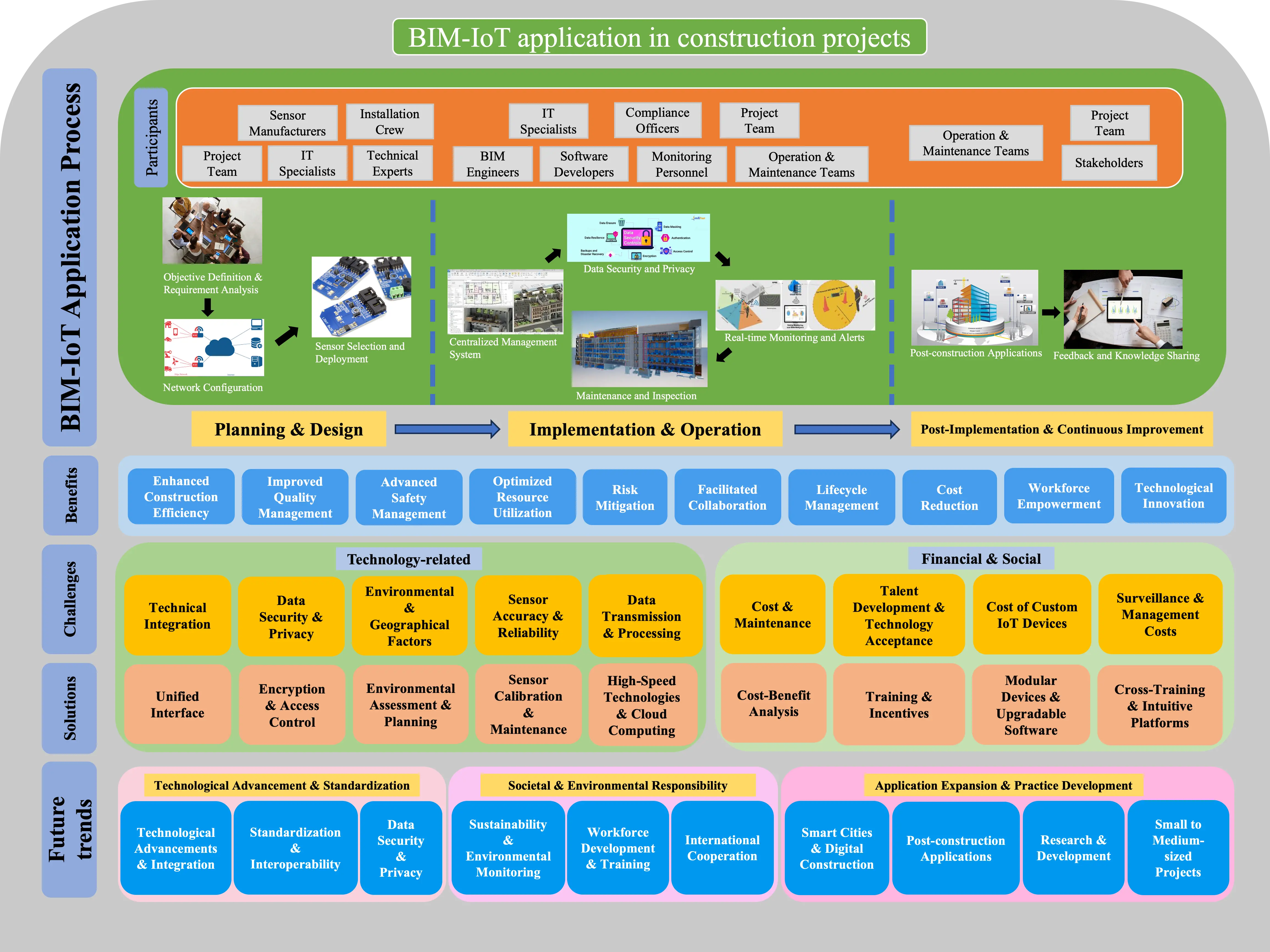
Figure 6. Proposed BIM-IoT application framework in construction projects. BIM: Building Information Modeling; IoT: Internet of Things.
5.1 BIM-IoT application process
Based on the individual case studies analyzed, the general process for using BIM-IoT in construction projects is summarized as follows:
1. Objective Definition: The project team should first define the goals of using BIM-IoT, such as improving construction efficiency, resource optimization, and safety management.
Participants : Project Team.
2. Requirement Analysis: Relevant experts and project personnel need to conduct in-depth analysis of the project to determine the key parameters that need to be monitored. This step requires determining the type, number and installation location of the sensors required.
Participants : Technical Experts, Project Team.
3. Network Configuration: The sensor network layout is carried out by technical experts according to the specific requirements of the project and the parameters that need to be monitored. In this step, it is necessary to determine the communication method and communication protocol of data transmission.
Participants : IT Specialists.
4. Sensor Selection and Deployment: Relevant personnel should choose the appropriate installation equipment according to the needs of the project, and install the sensor in the specific part of the site.
Participants : Sensor Manufacturers, Installation Crew, Project Team.
5. Centralized Management System: BIM engineers connect sensors on the site to an online platform developed specifically for the project to receive, store and analyze the data collected by the sensors. The system should ensure the reliability of the processing results.
Participants : BIM Engineers, Software Developers.
6. Data Security and Privacy: The BIM team implements data security measures, including data encryption, access control, backup and disaster recovery. Compliance Officers will conduct compliance audits and monitoring to ensure data integrity and traceability.
Participants : IT Specialists, Compliance Officers.
7. Real-time Monitoring and Alerts: IoT technology enables real-time data collection and monitoring on construction sites. During the construction phase, managers can identify and deal with construction risks in a timely manner through the BIM platform to strengthen safety management.
Participants : Monitoring Personnel, Project Team.
8. Maintenance and Inspection: The management team regularly maintains and checks the sensor equipment during the construction phase to ensure the normal operation of the sensor equipment and the accuracy of the collected data. After the construction of the building is completed, the operations team takes over the platform, and the sensors also need to be checked and maintained regularly.
Participants : Operation and Maintenance Teams.
9. Post-construction Applications: The BIM model is also used for post-construction operations planning, and after taking over the BIM-IoT platform, the operations team can obtain detailed asset information and guidance.
Participants : Operation and Maintenance Teams.
10. Feedback and Knowledge Sharing: Project teams get feedback and share knowledge to improve BIM-IoT processes in future projects.
Participants : Project Team, Stakeholders.
5.2 Benefits of using BIM-IoT
After outlining the general process of BIM-IoT integration, the main benefits of using BIM-IoT in construction projects can be summarized as follows:
Enhanced Construction Efficiency: BIM-IoT enables real-time monitoring and management of the construction process, simplifying operations and improving overall efficiency.
Improved Quality Management: By integrating BIM with IoT, the construction team can monitor key parameters such as environmental conditions and ensure quality control throughout the project.
Advanced Safety Management: IoT sensors can detect potential security hazards in real time, when integratedwith BIM, they can provide instant alerts to potential security hazards and allow for rapid responses, reducing risks to workers and projects.
Optimized Resource Utilization: BIM-IoT helps to better plan and allocate resources such as manpower, equipment and materials, potentially reducing logistics costs and time.
Risk Mitigation: BIM-IoT systems can identify and alert project teams to potential risks, allowing proactive action to be taken before they escalate into bigger problems.
Facilitated Collaboration: BIM-IoT technology can improve communication and collaboration among project stakeholders by providing a centralized platform for data sharing and visualization.
Lifecycle Management: BIM-IoT applications extend from the construction phase to the operations and maintenance phase, providing valuable data for asset management and life cycle planning.
Cost Reduction: By improving efficiency, quality control and risk management, BIM-IoT can reduce costs throughout the life cycle of a construction project.
Workforce Empowerment: Providing real-time data and insights to construction workers empowers them with the information needed to make better operational decisions on site.
Technological Innovation: The adoption of BIM-IoT technology can drive innovation in the construction industry, encouraging the development and use of advanced tools and methods.
5.3 Common challenges of using BIM-IoT and mitigation solutions
By analyzing the three case studies, though the benefits of using BIM-IoT are evident, there are also common challenges that have been observed and summarized below along with the corresponding mitigation solutions:
Technical Integration: Compatibility and integration issues between different BIM and IoT systems can result in data not being communicated, affecting the efficiency of communication between parties. The project team needs to communicate with the manufacturers, maintain a unified interface import platform for data communication, ensure the integration of sensor data with the BIM platform, and maintain the consistency of data formats and communication protocols.
Data Security and Privacy: Given the large number of people involved in the construction project, the data of the sensor and the privacy of the project are easy to leak. To mitigate this risk, projects need to adopt encryption technologies, implement access control policies, and conduct regular data security audits to prevent unauthorized access and data breaches.
Environmental and Geographical Factors: Extreme weather conditions and difficult terrain can threaten the stability of construction equipment and sensors. Project teams are required to conduct environmental impact assessments, select equipment and sensors suitable for local environmental conditions, and develop contingency plans for extreme weather events.
Sensor Accuracy and Reliability: The accuracy and stability of the sensors directly affect the data quality and effectiveness of the monitoring system. To ensure accurate data, the project team should select mature sensor brands and regularly calibrate and maintain the sensors
Cost and Maintenance: The economic burden of procuring, deploying, and maintaining sensors can be significant. It is necessary to develop a cost-benefit analysis, optimized sensor deployment plans, cost-effective solutions, and long-term maintenance and replacement plans for the project.
Data Transmission and Processing: The transmission and processing of large amounts of real-time data requires efficient network and computing resources. The project should utilize high-speed data transmission technologies such as 5G or fiber optic communication, and utilize cloud and edge computing technologies for data processing and analysis.
Talent Development and Technology Acceptance: Insufficient employee knowledge and trust in BIM and IoT technologies can hinder the adoption and effective use of technologies. The program needs to invest in employee training and education to increase their awareness and operational capabilities of the technologies, and create incentives to encourage the use of the relevant technologies and tools.
Cost of Custom IoT Devices: Tailoring IoT devices to the specific requirements of a construction project can incur significant expenses. The specificity of these devices often limits their reuse, which can be seen as an inefficient allocation of resources. To offset the high cost of customization, building entities can opt for modular IoT devices that can be utilized multiple times in multiple projects without incurring excessive costs.
Surveillance and Management Costs: The implementation of IoT technology is expected to improve operational efficiency, but it may also lead to increased monitoring and management expenses. Skilled personnel are needed to oversee IoT operations as well as data processing, which can increase the cost of the project. Companies can implement cross-training programs for existing employees to gain a broader understanding and management of IoT systems. Leveraging intuitive IoT platforms that require minimal expertise can also help reduce reliance on professionals.
5.4 Future trends of BIM-IoT in China
In general, the implementation of BIM-IoT has a profound impact on construction projects and will be continuously improved to better meet the industry demand and to facilitate a smarter construction industry. Based on the interview results of this study, the future trends of BIM-IoT in the construction industry in China are very likely to reside in the following aspects. The future of BIM-IoT in construction projects in China will usher in significant growth and innovation. Technological advances will lead to tighter integration with artificial intelligence and machine learning, creating smarter construction management systems. The government's standardization efforts will enhance interoperability between different systems, while the focus on sustainability will leverage BIM-IoT for environmental monitoring. As these technologies become more entrenched in project management, data security will become critical. Workforce development will be the key to the successful adoption of these advanced technologies, and BIM-IoT is expected to become widespread in small projects as costs decrease. The smart city trend will increasingly incorporate BIM-IoT into urban development, and post-construction applications will become more prevalent in asset and lifecycle management. Continued investment in research and development and international collaboration will drive technological advances, facilitate the widespread adoption of BIM-IoT in the construction industry in China.
6. Conclusion
6.1 Key findings and contributions
This paper investigates the application of BIM-IoT in the construction projects in China, and the results show that BIM-IoT plays a key role in improving building efficiency, quality management, and resource optimization. Through a comprehensive multi-case study approach, the study presents the application process of BIM-IoT in construction projects, highlights the transformative impact of BIM-IoT on construction practice, and proposes solutions to main challenges of BIM-IoT. The results of the study provide a clear roadmap for the implementation of BIM-IoT in construction projects and its future development in the construction industry.
The study concludes that the convergence of BIM and IoT marks an important step towards a smarter and safer approach to construction, which is consistent with the goal of sustainable urban development in China. Moreover, this study proposes a BIM-IoT application framework based on the results obtained from the multiple case studies. Through the collaborative application of this framework, BIM-IoT can significantly improve construction efficiency, strengthen quality control, and optimize resource allocation. While emphasizing the benefits of BIM-IoT, the framework also recognizes and addresses the inherent challenges of BIM-IoT, such as technology integration, data security, and privacy issues.
With the identified challenges of BIM-IoT and the proposed solutions, the study highlights the importance of high-speed networks, cloud platforms, and cross-training to develop the skills employees to effectively manage and operate complex BIM-IoT systems. It emphasizes the need for intuitive interfaces, modular devices, and upgradeable software to facilitate technological change. The study also foresees the convergence of BIM-IoT with smart city and digital construction initiatives. It emphasizes the importance of standardization and interoperability for maintaining systems and environmental sustainability in the long term.
As the construction industry continues to evolve, the insights gained from this paper will serve as a valuable reference for construction project teams in their application of BIM-IoT, ensuring that the industry can meet the demands of sustainable development and technological innovation head-on.
6.2 Research limitations and future research directions
While this study provides a comprehensive examination of BIM-IoT applications, certain limitations are acknowledged. Firstly, the study's scope, though broad, is confined to the limited number of case studies, which may not fully represent the diversity of construction projects. Secondly, the dynamic nature of technology evolution implies that emerging tools and methodologies not covered in this research could offer additional insights. Thirdly, all case studies were conducted in China, which might include the influence of culturally specific organizational practices and the unique regulatory environment. As highlighted by Rahimian et al.[29], barriers such as political and regulatory constraints, cybersecurity risks, and the lack of standardized frameworks pose significant challenges to IoT adoption in construction. In regions with stringent data protection laws, such as the EU and North America, additional security measures and compliance protocols may be necessary. Furthermore, market readiness and financial investment in digital construction vary, impacting the feasibility of large-scale BIM-IoT adoption. To enhance international applicability, the framework should be adapted to align with local regulatory policies, workforce expertise, and technological infrastructure.
Future studies could include a wider range of construction projects to provide a more holistic view of BIM-IoT applications across different types of projects. The absence of an international comparative perspective in the study further underscores the necessity for broader research to fully appreciate how BIM-IoT can be adapted to various global contexts. To further validate the proposed framework, future research should conduct large-scale empirical testing across diverse construction environments. This could include pilot projects in different geographic regions, incorporating varied regulatory, economic, and cultural conditions. A comparative analysis between developed and developing markets would provide insights into the adaptability of the framework under different constraints. Additionally, quantitative assessments, such as performance metrics and cost-benefit analyses, could be used to evaluate the practical impact of BIM-IoT implementation. Expanding the sample size by engaging with a broader range of industry stakeholders, including contractors, regulatory bodies, and technology providers, would enhance the framework's reliability and ensure its effectiveness in guiding BIM-IoT adoption on a global scale.
Supplementary materials
The supplementary material for this article is available at: Supplementary materials.
Acknowledgments
The authors would like to sincerely express their gratitude for the support provided by the practitioners who participated in this project.
Authors contribution
Guo F: Article conception and design, substantive revision of the manuscript.
Hong Y: Article conception and design, data analysis and interpretation, substantive revision of the manuscript.
All authors approved the final version of the manuscript.
Conflicts of interest
Dr. Fangyu Guo received financial support from the Glodon company.
Ethical approval
This research has obtained the approval from our university research ethics review panel with the document No. ER-LRR-0010000037820240126153250.
Consent to participate
Informed consent was obtained from all participants.
Consent for publication
Not applicable
Availability of data and materials
Not applicable
Funding
This article was supported by the research project RDS10120220066 from Glodon Company.
Copyright
© The Author(s) 2025.
References
-
1. Tang S, Shelden DR, Eastman CM, Pishdad-Bozorgi P, Gao X. A review of building information modeling (BIM) and the internet of things (IoT) devices integration: Present status and future trends. Autom Constr. 2019;101:127-139.
[DOI] -
2. Zhou Y, Yang Y, Yang JB. Barriers to BIM implementation strategies in China. Eng Constr Archit Manag. 2019;26(3):554-574.
[DOI] -
3. Olanrewaju OI, Kineber AF, Chileshe N, Edwards DJ. Modelling the relationship between Building Information Modelling (BIM) implementation barriers, usage and awareness on building project lifecycle. Build Environ. 2022;207(Part B):108556.
[DOI] -
4. Song X, Song J. Analysis on the application of BIM in design mode. Constr Superv. 2020;07:13-15. Chinese.
[DOI] -
5. Yevu SK, Ann T, Darko A. Digitalization of construction supply chain and procurement in the built environment: Emerging technologies and opportunities for sustainable processes. J Clean Prod. 2021;322:129093.
[DOI] -
6. Tagliabue LC, Ciribini ALC. A BIM-Based IoT Approach to the Construction Site Management. In_bo. 2018;9(13):136-145.
[DOI] -
7. Humayun M, Alsaqer MS, Jhanjhi N. Energy optimization for smart cities using iot. Appl Artif Intell. 2022;36(1):2037255.
[DOI] -
8. Zhou JX, Shen GQ, Yoon SH, Jin X. Customization of on-site assembly services by integrating the internet of things and BIM technologies in modular integrated construction. Autom Constr. 2021;129:103663.
[DOI] -
9. Zhao Y, Cao C, Liu Z, Mu E. Intelligent control method of hoisting prefabricated components based on internet-of-things. Sensors. 2021;21(3):980.
[DOI] -
10. Wu L, Lu W, Xue F, Li X, Zhao R, Tang M. Linking permissioned blockchain to Internet of Things (IoT)-BIM platform for off-site production management in modular construction. Comput Ind. 2022;135:103573.
[DOI] -
11. Wang X, Wang S, Song X, Han Y. IoT-based intelligent construction system for prefabricated buildings: Study of operating mechanism and implementation in China. Appl Sci. 2020;10(18):6311.
[DOI] -
12. Aryal A, Ghahramani A, Becerik-Gerber B. Monitoring fatigue in construction workers using physiological measurements. Autom Constr. 2017;82:154-165.
[DOI] -
13. Hwang S, Lee S. Wristband-type wearable health devices to measure construction workers' physical demands. Autom Constr. 2017;83:330-340.
[DOI] -
14. Chung WWS, Tariq S, Mohandes SR, Zayed T. IoT-based application for construction site safety monitoring. Int J Constr Manag. 2020;23(1):58-74.
[DOI] -
15. Lee UK, Kim JH, Cho H, Kang KI. Development of a mobile safety monitoring system for construction sites. Autom Constr. 2009;18(3):258-264.
[DOI] -
16. Joshua L, Varghese K. Automated recognition of construction labour activity using accelerometers in field situations. Int J Product Perform Manag. 2014;63(7):841-862.
[DOI] -
17. Nath ND, Akhavian R, Behzadan AH. Ergonomic analysis of construction worker's body postures using wearable mobile sensors. Appl Ergon. 2017;62:107-117.
[DOI] -
18. Xiahou X, Wu Y, Duan T, Lin P, Li F, Qu X, et al. Analyzing critical factors for the smart construction site development: A DEMATEL-ISM based approach. Buildings. 2022;12:116.
[DOI] -
19. Nguyen HA, Nguyen LV, Ha QP. IoT-enabled dependable co-located low-cost sensing for construction site monitoring. In: 2020 Proceedings of the 37th ISARC; 2020 Oct 27-28; Kitakyushu, Japan. Vienna: IAARC; 2020. p. 616-624.
[DOI] -
20. Touqeer H, Zaman S, Amin R, Hussain M, Al-Turjman F, Bilal M. Smart home security: challenges, issues and solutions at different IoT layers. J Supercomput. 2021;77:14053-14089.
[DOI] -
21. Stolojescu-Crisan C, Crisan C, Butunoi BP. An IoT-based smart home automation system. Sensors. 2021;21(11):3784.
[DOI] -
22. Guida CG, Gupta BB, Lorusso A, Marongiu F, Santaniello D, Troiano A. An Integrated BIM-IoT approach to support energy monitoring. In: Nedjah N, Perez GM, Gupta BB, editors. International Conference on Smart Systems and Advanced Computing (SysCom 2022). Germany: CEUR Workshop Proceedings; 2021.
-
23. Scianna A, Gaglio GF, Guardia ML. Structure monitoring with BIM and IoT: The case study of a bridge beam model. ISPRS Int J Geo-Inf. 2022;11(3):173.
[DOI] -
24. Hancock DR, Algozzine B, Lim JH. Doing case study research: A practical guide for beginning researchers. United States: SAGE Publications; 2021.
-
25. Savin-Baden M, Major CH. Qualitative research: The essential guide to theory and practice. London: Routledge; 2023.
-
26. Adeoye-Olatunde OA, Olenik NL. Research and scholarly methods: Semi-structured interviews. J Am Coll Clin Pharm. 2021;4(10):1358-1367.
-
27. Limaj E, Bernroider EWN. A systematic analysis and synthesis of case study based agile scaling research in the context of digital transformations. In: Doucek P, Basl J, Tjoa A, Raffai M, Pavlicek A, Detter K, editors. Research and Practical Issues of Enterprise Information Systems; 2019 Dec 16-17; Prague, Czech Republic. Cham: Springer; 2019. p. 74-84.
[DOI] -
28. Dardouri S, BuHamdan S, Al Balkhy, Dakhli Z, Danel T, Lafhaj Z. RFID platform for construction materials management. Int J Constr Manag. 2023;23(14):2509-2519.
[DOI] -
29. Rahimian A, Ahmed RR, Fazeli A, Aghdam E, Singh AK, Naji M, et al. Examining the Barriers to the Adoption of IoT-Based Technologies in Green Buildings. J Constr Eng Manag. 2024;151(3):04024211.
[DOI]
Copyright
© The Author(s) 2025. This is an Open Access article licensed under a Creative Commons Attribution 4.0 International License (https://creativecommons.org/licenses/by/4.0/), which permits unrestricted use, sharing, adaptation, distribution and reproduction in any medium or format, for any purpose, even commercially, as long as you give appropriate credit to the original author(s) and the source, provide a link to the Creative Commons license, and indicate if changes were made.
Share And Cite